Taking advantage of detectors used for particle physics, cosmogenic muons are becoming powerful tools for non-destructive imaging of large structures such as pyramids. Physicist and muographer Andrea Giammanco explains.
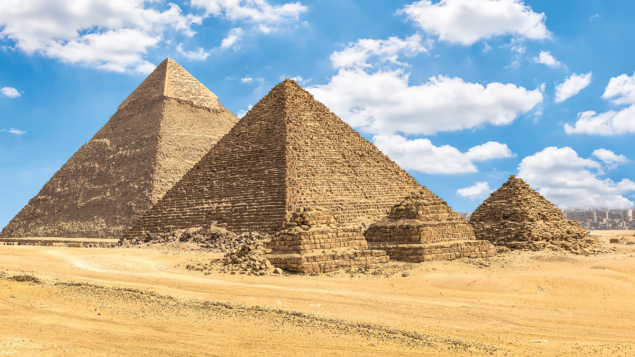
In 1965, three years before being awarded a Nobel prize for his decisive contributions to elementary particle physics, Luis Alvarez proposed to use cosmic muons to look inside an Egyptian pyramid. A visit to the Giza pyramid complex a few years earlier had made him ponder why, despite the comparable size of the Great Pyramid of Khufu and the Pyramid of Khafre, the latter was built with a simpler structure – simpler even than the tomb of Khufu’s great-grandfather Sneferu, under whose reign there had been architectural experimentation and pyramids had grown in complexity. Only one burial chamber is known in the superstructure of Khafre’s pyramid, while two are located in the tombs of each of his two predecessors. Alvarez’s doubts were not shared by many archaeologists, and he was certainly aware that the history of architecture is not a continuous process and that family relationships can be complicated; but like many adventurers before him, he was fascinated by the idea that some hidden chambers could still be waiting to be discovered.
The principles of muon radiography or “muography” were already textbook knowledge at that time. Muons are copiously produced in particle cascades originating from naturally occurring interactions between primary cosmic rays and atmospheric nuclei. The energy of most of those cosmogenic muons is large enough that, despite their relatively short intrinsic lifetime, relativistic dilation allows most of them to survive the journey from the upper atmosphere to Earth’s surface – where their penetration power makes them a promising tool to probe the depths of very large and dense volumes non-destructively. Thick and dense objects can attenuate the cosmic-muon flux significantly by stopping its low-energy component, thus providing a “shadow” analogous to conventional radiographies. The earliest known attempt to use the muon flux attenuation for practical purposes was the estimation of the overburden of a tunnel in Australia using Geiger counters on a rail, published in 1955 in an engineering journal. The obscure precedent was probably unknown to Alvarez, who didn’t cite it.
Led by Alvarez, the Joint Pyramid Project was officially established in 1966. The detector that the team built and installed in the known large chamber at the bottom of Khafre’s pyramid was based on spark chambers, which were standard equipment for particle-physics experiments at that time. Less common were the computers provided by IBM for Monte Carlo simulations, which played a crucial role in the data interpretation. It took some time for the project to take off. Just as the experiment was ready to take data, the Six-Day War broke out, delaying progress by several months until diplomatic relationships were restored between Cairo and Washington. All this might sound like a promising subject for a Hollywood blockbuster were it not for its anticlimax: no hidden chamber was found. Alvarez always insisted that there is a difference between not finding what you search for and conclusively excluding its existence, but despite this important distinction, one wonders how much muography’s fame would have benefitted from a discovery. Their study, published in Science in 1970, set an example that was followed in subsequent decades by many more interdisciplinary applications.
The second pyramid to be muographed was in Mexico more than 30 years later, when researchers from the National Autonomous University of Mexico (UNAM) started to search for hidden chambers in the Pyramid of the Sun at Teotihuacan. Built by the Aztecs about 1800 years ago, it is the third largest pyramid in the world after Khufu’s and Khafre’s, and its purpose is still a mystery. Although there is no sign that it contains burial chambers, the hypothesis that this monument served as a tomb is not entirely ruled out. After more than a decade of data taking, the UNAM muon detector (composed of six layers of multi-wire chambers occupying a total volume of 1.5 m3) found no hidden chamber. But the researchers did find evidence, reported in 2013, for a very wide low-density volume in the southern side, which is still not understood and led to speculation that this side of the pyramid might be in danger of collapse.
Big void
Muography returned to Egypt with the ScanPyramids project, which has been taking data since 2015. The project made the headlines in 2017 by revealing an unexpected low-density anomaly in Khufu’s Great Pyramid, tantalisingly similar in size and shape to the Grand Gallery of the same building. Three teams of physicists from Japan and France participated in the endeavour, cross-checking each other by using different detector technologies: nuclear emulsions, plastic scintillators and micromegas. The latter, being gaseous detectors, had to be located externally to the pyramid to comply with safety regulations. Publishing in Nature Physics, all three teams reported a statistically significant excess in muon flux originating from the same 3D position (see “Khufu’s pyramid” figure).
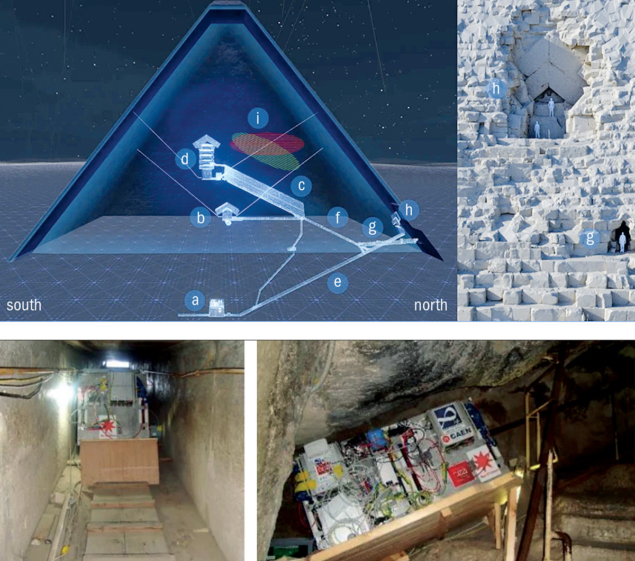
This year, based on a larger data sample, the ScanPyramids team concluded that this “Big Void” is a horizontal corridor about 9 m long with a transverse section of around 2 × 2 m2. Confidence in the solidity of these conclusions was provided by a cross-check measurement with ground-penetrating radar and ultrasounds, by Egyptian and German experts, which took data since 2020 and was published simultaneously. The consistency of the data from muography and conventional methods motivated visual inspection via an endoscope, confirming the claim. While the purpose of this unexpected feature of the pyramid is not yet known, the work represents the first characterisation of the position and dimensions of a void detected by cosmic-ray muons with a sensitivity of a few centimetres.
New projects exploring the Giza pyramids are now sprouting. A particularly ambitious project by researchers in Egypt, the US and the UK – Exploring the Great Pyramid (EGP) – uses movable large-area detectors to perform precise 3D tomography of the pyramid. Thanks to its larger surface and some methodological improvements, EGP aims to surpass ScanPyramids’ sensitivity after two years of data taking. Although still at the simulation studies stage, the detector technology – plastic scintillator bars with a triangular section and encapsulated wavelength shifter fibres – is already being used by the ongoing MURAVES muography project to scan the interior of the Vesuvius volcano in Italy. The project will also profit from synergy with the upcoming Mu2e experiment at Fermilab, where the very same detectors are used. Finally, proponents of the ScIDEP (Scintillator Imaging Detector for the Egyptian Pyramids) experiment from Egypt, the US and Belgium are giving Khafre’s pyramid a second look, using a high-resolution scintillator-based detector to take data from the same location as Alvarez’s spark chambers.
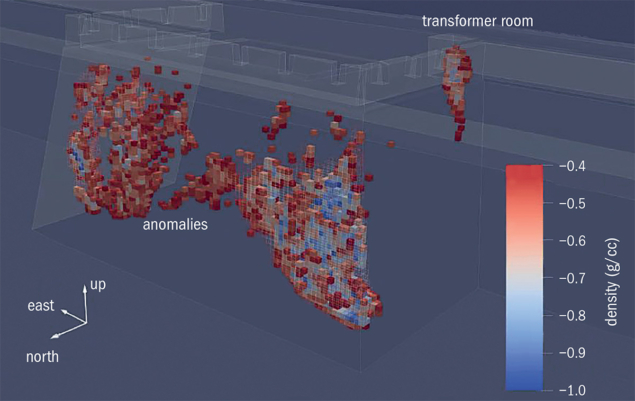
Pyramids easily make headlines, but there is no scarcity of monuments around the world where muography can play a role. Recently, a Russian team used emulsion detectors to explore the Svyato–Troitsky Danilov Monastery, the main buildings of which have undergone several renovations across the centuries but with associated documentation lost. The results of their survey, published in 2022, include evidence for two unknown rooms and areas of significantly higher density (possible walls) in the immured parts of certain vaults, and of underground voids speculated to be ancient crypts or air ducts. Muography is also being used to preserve buildings of historical importance. The defensive wall structures of Xi’an, one of the Four Great Ancient Capitals of China, suffered serious damage due to heavy rainfall, but repairs in the 1980s were insufficiently documented, motivating non-destructive techniques to assess their internal status. Taking data from six different locations using a compact and portable muon detector to extract a 3D density map of a rampart, a Chinese team led by Lanzhou University has recently reported density anomalies that potentially pose safety hazards (see “Falling walls” figure).
The many flavours of muography
All the examples described so far are based on the same basic principle as Alvarez’s experiment: the attenuation of the muon flux through dense matter. But there are other ways to utilise muons as probes. For example, it is possible to exploit their deflection in matter due to Coulomb scattering from nuclei, offering the possibility of elemental discrimination. Such muon scattering tomography (MST) has been proposed to help preserve the Santa Maria del Fiore cathedral in Florence, built between 1420 and 1436 by Filippo Brunelleschi, the iconic dome of which is cracking under its own weight. Accurate modelling is needed to guide reinforcement efforts, but uncertainties exist on the internal structure of the walls. According to some experts, Brunelleschi might have inserted iron chains inside the masonry of the dome to stabilise it; however, no conclusive evidence has been obtained with traditional remote-sensing methods. Searching for iron within masonry is therefore the goal of the proposed experiment (see “Preserving a masterpiece” figure), for which a proof-of-principle test on a mock-up wall has already been carried out in Los Alamos.
Beyond cultural heritage, muography has also been advocated as a powerful remote-sensing method for a variety of applications in the nuclear sector. It has been used, for example, to assess the damage and impact of radioactivity in the Fukushima power plant, where four nuclear reactors were damaged in 2011. Absorption-based muography was applied to determine the difference in the density, for example the thickness of the walls, within the nuclear reactor while MST was applied to locate the nuclear fuel. Muography, especially MST, has allowed the investigation of other extreme systems, including blast furnaces and nuclear waste barrels.
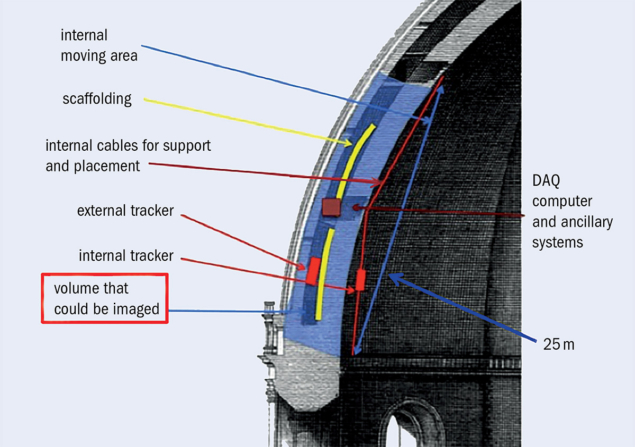
Volcanology is a further important application of muography, where it is used to discover empty magma chambers and voids. As muons are better absorbed by thick and dense objects, such as rocks on the bottom of a volcano, the absorption provides key information about its inner structure. The density images created via muography can even be fed into machine-learning models to help predict eruptive patterns, and similar methods can be applied to glaciology, as has been done to estimate the topography of mountains hidden by overlaying glaciers. Among these projects is Eiger-μ, designed to explore the mechanisms of glacial erosion.
Powerful partnership
Muography creates bridges across the world between particle physics and cultural-heritage preservation. The ability to perform radiography of a large object from a distance or from pre-existing tunnels is very appealing in situations where invasive excavations are impossible, as is often the case in highly populated urban or severely constrained areas. Geophysical remote-sensing methods are already part of the archaeological toolkit, but in general they are expensive, have a limited resolution and demand strong model assumptions for interpreting the data. Muography is now gaining acceptance in the cultural-heritage preservation world because its data are intrinsically directional and can be easily interpreted in terms of density distributions.
From the pioneering work of Alvarez to the state-of-the-art systems available today, progress in muography has gone hand-in-hand with the development of detectors for particle physics. The ScanPyramids project, for example, uses micropattern gaseous detectors such as those developed within the CERN RD51 collaboration and nuclear emulsion detectors as those of the OPERA neutrino experiment, while the upcoming EGP project will benefit from detector technologies for the Mu2e experiment at Fermilab. R&D for next-generation muography includes the development of scintillator-based muon detectors, resistive plate chambers, trackers based on multi-wire proportional chambers and more. There are proposals to use microstrip silicon detectors from the CMS experiment and Cherenkov telescopes inspired by the CTA astrophysics project, showing how R&D for fundamental physics continues to drive exotic applications in archaeology and cultural-heritage preservation.
Further reading
A B Alexandrov et al. 2022 Phys. Part. Nucl. 53 1146.
L W Alvarez et al. 1970 Science 167 832.
L Bonechi et al. 2020 Rev. Phys. 5 100038.
A Bross et al. 2022 JAIS 280.
E Guardincerri et al. 2018 Philos. Trans. R. Soc. A 377 0136.
IAEA 2022 IAEA-TECDOC-2012.
G Liu et al. 2023 J. Appl. Phys.133 014901.
K Morishima et al. 2017 Nature 552 386.
L Olah et al. (eds) 2022 Muography: Exploring Earth’s Subsurface with Elementary Particles (Wiley).
S Procureur et al. 2023 Nat. Commun. 14 1144.