The brand-new “SciFi” tracker and upgraded ring-imaging Cherenkov detectors that are currently being installed are vital for the higher LHC luminosities ahead, write Christoph Frei, Silvia Gambetta and Blake Leverington.
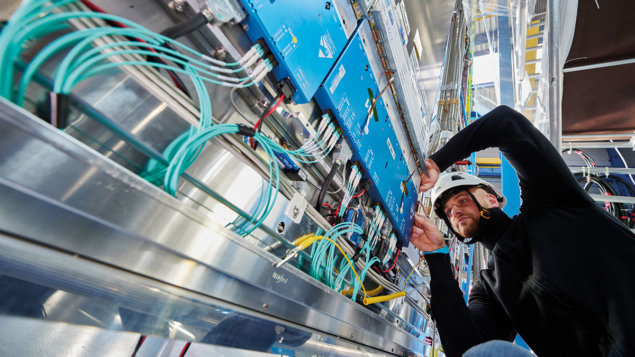
It was once questioned whether it would be possible to successfully operate an asymmetric “forward” detector at a hadron collider. In such a high-occupancy environment, it is much harder to reconstruct decay vertices and tracks than it is at a lepton collider. Following its successes during LHC Run 1 and Run 2, however, LHCb has rewritten the forward-physics rulebook, and is now preparing to take on bigger challenges.
During Long Shutdown 2, which comes to an end early next year, the LHCb detector is being almost entirely rebuilt to allow data to be collected at a rate up to 10 times higher during Run 3 and Run 4. This will improve the precision of numerous world-best results, such as constraints on the angles of the CKM triangle, while further scrutinising intriguing results in B-meson decays, which hint at departures from the Standard Model.

At the core of the LHCb upgrade project are new detectors capable of sustaining an instantaneous luminosity up to five times that seen at Run 2, and which enable a pioneering software-only trigger that will enable LHCb to process signal data in an upgraded computing farm at the frenetic rate of 40 MHz. The vertex locator (VELO) will be replaced with a pixel version, the upstream silicon-strip tracker will be replaced with a lighter version (the UT) located closer to the beamline, and the electronics for LHCb’s muon stations and calorimeters are being upgraded for 40 MHz readout.
Recently, three further detector systems key to dealing with the higher occupancies ahead were lowered into the LHCb cavern for installation: the upgraded ring-imaging Cherenkov detectors RICH1 and RICH2 for sharper particle identification, and the brand new “SciFi” (scintillating fibre) tracker.
SciFi tracking
The components of LHCb’s SciFi tracker may not seem futuristic at first glance. Its core elements are constructed from what is essentially paper, plastic, some carbon fibre and glue. However, its materials components conceal advanced technologies which, when coupled together, produce a very light and uniform, high-performance detector that is needed to cope with the higher number of particle tracks expected during Run 3.
Located behind the LHCb magnet (see “Asymmetric anatomy” image), the SciFi represents a challenge, not only due to its complexity, but also because the technology – plastic scintillating fibres and silicon photomultiplier arrays – has never been used for such a large area in such a harsh radiation environment. Many of the underlying technologies have been pushed to the extreme during the past decade to allow the SciFi to successfully operate under LHC conditions in an affordable and effective way.
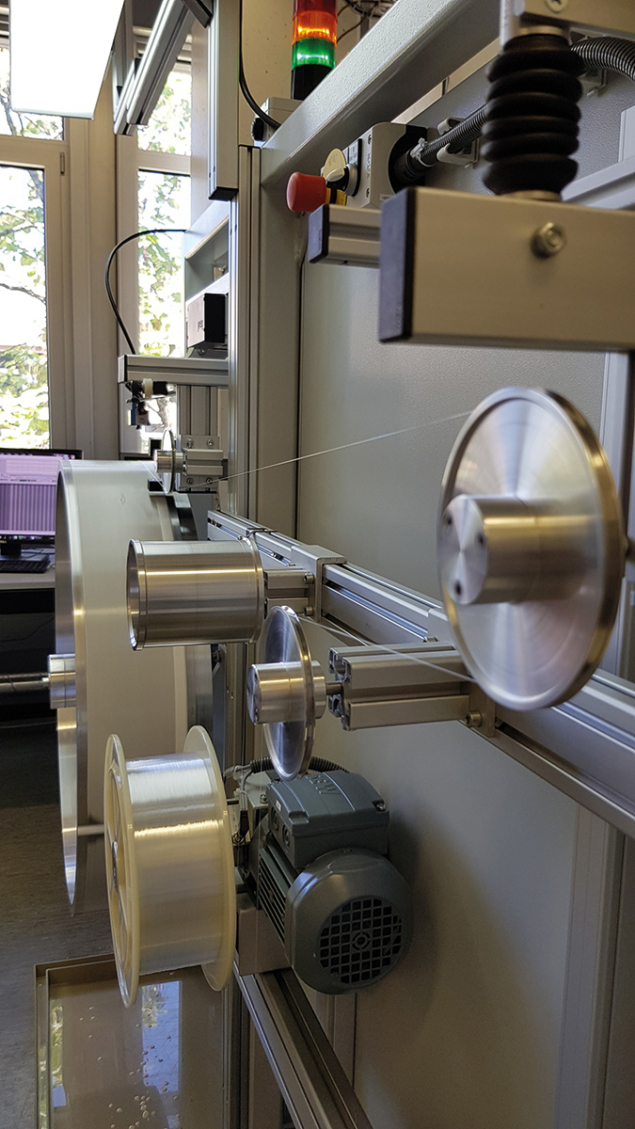
More than 11,000 km of 0.25 mm-diameter polystyrene fibre was delivered to CERN before undergoing meticulous quality checks. Excessive diameter variations were removed to prevent disruptions of the closely packed fibre matrix produced during the winding procedure, and clear improvements from the early batches to the production phase were made by working closely with the industrial manufacturer. From the raw fibres, nearly 1400 multi-layered fibre mats were wound in four of the LHCb collaboration’s institutes (see “SciFi spools” image), before being cut and bonded in modules, tested, and shipped to CERN where they were assembled with the cold boxes. The SciFi tracker contains 128 stiff and robust 5 × 0.5 m2 modules made of eight mats bonded with two fire-resistant honeycomb and carbon-fibre panels, along with some mechanics and a light-injection system. In total, the design produces nearly 320 m2 of detector surface over the 12 layers of the tracking stations.
The scintillating fibres emit photons at blue-green wavelengths when a particle interacts with them. Secondary scintillator dyes added to the polystyrene amplify the light and shift it to longer wavelengths so it can be read out by custom-made silicon photomultipliers (SiPMs). SiPMs have become a strong alternative to conventional photomultiplier tubes in recent years, due to their smaller channel sizes, easier operation and insensitivity to magnetic fields. This makes them ideal to read out the higher number of channels necessary to identify separate but nearby tracks in LHCb during Run 3.
The width of the SiPM channels, 0.25 mm, is designed to match that of the fibres. Though they need not align perfectly, this provides a better separation power for tracking than the previously used 5 mm gas straw tubes in the outer regions of the detector, while providing a similar performance to the silicon-strip tracker. The tiny channel size results in over 524,288 SiPM channels to collect light from 130 m of fibre-mat edges. A custom ASIC, called the PACIFIC, outputs two bits per channel based on three signal-amplitude thresholds. A field-programmable gate array (FPGA) assigned to each SiPM then groups these signals into clusters, where the location of each cluster is sent to the computing farm. Despite clustering and noise suppression, this still results in an enormous data rate of 20 Tb/s – nearly half of the total data bandwidth of the upgraded LHCb detector.
One of the key factors in the success of LHCb’s flavour-physics programme is its ability to identify charged particles
LHCb’s SciFi tracker is the first large-scale use of SiPMs for tracking, and takes advantage of improvements in the technology in the 10 years since the SciFi was proposed. The photon-detection efficiency of SiPMs has nearly doubled thanks to improvements in the design and production of the underlying pixel structures, while the probability of crosstalk between the pixels (which creates multiple fake signals by causing a single pixel to randomly fire without incident light following radiation damage) has been reduced from more than 20% to a few percent by the introduction of microscopic trenches between the pixels. The dark-single-pixel firing rate can also be reduced by cooling the SiPM. Together, these two methods greatly reduce the number of fake-signal clusters such that the tracker can effectively function after several years of operation in the LHCb cavern.
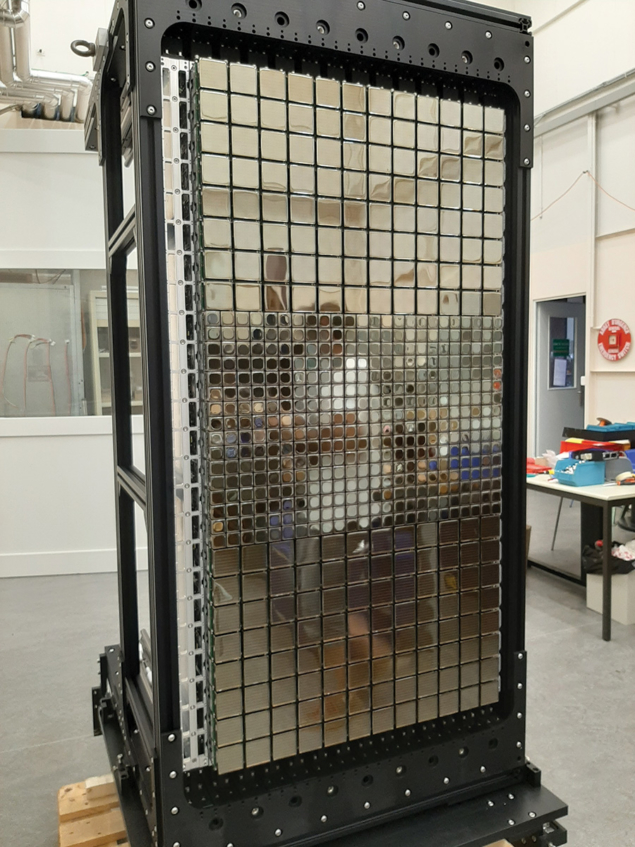
The LHCb collaboration assembled commercial SiPMs on flex cables and bonded them in groups of 16 to a 0.5 m-long 3D-printed titanium cooling bar to form precisely assembled photodetection units for the SciFi modules. By circulating a coolant at a temperature of –50 °C through the cold bar, the dark-noise rate was reduced by a factor of 60. Furthermore, in a first for a CERN experiment, it was decided to use a new single-phase liquid coolant called Novec-649 from 3M for its non-toxic properties and low greenhouse warming potential (GWP = 1). Historically, C6F14 – which has a GWP = 7400 – was the thermo-transfer fluid of choice. Although several challenges had to be faced in learning how to work with the new fluid, wider use of Novec-649 and similar products could contribute significantly to the reduction of CERN’s carbon footprint. Additionally, since the narrow envelope of the tracking stations precludes the use of standard foam insulation of the coolant lines, a significant engineering effort has been required to vacuum insulate the 48 transfer lines from the 24 rows of SiPMs and 256 cold-bars where leaks are possible at every connection.
To date, LHCb collaborators have tirelessly assembled and tested nearly half of the SciFi tracker above ground, where only two defective channels out of the 262,144 tested in the full signal chain were unrecoverable. Four out of 12 “C-frames” containing the fibre modules (see “Tracking tall” image) are now installed and waiting to be connected and commissioned, with a further two installed in mid-July. The remaining six will be completed and installed before the start of operations early next year.
New riches
One of the key factors in the success of LHCb’s flavour-physics programme is its ability to identify charged particles, which reduces the background in selected final states and assists in the flavour tagging of b quarks. Two ring-imaging Cherenkov (RICH) detectors, RICH1 and RICH2, located upstream and downstream of the LHCb magnet 1 and 10 m away from the collision point, provide excellent particle identification over a very wide momentum range. They comprise a large volume of fluorocarbon gas (the radiator), in which photons are emitted by charged particles travelling at speeds higher than the speed of light in the gas; spherical and flat mirrors to focus and reflect this Cherenkov light; and two photon-detector planes where the Cherenkov rings are detected and read out by the front-end electronics.
The original RICH detectors are currently being refurbished to cope with the more challenging data-taking conditions of Run 3, requiring a variety of technological challenges to be overcome. The photon detection system, for example, has been redesigned to adapt to the highly non-uniform occupancy expected in the RICH system, running from an unprecedented peak occupancy of ~35% in the central region of RICH1 down to 5% in the peripheral region of RICH2. Two types of 64-channel multi-anode photomultiplier tubes (MaPMTs) have been selected for the task which, thanks to their exceptional quantum efficiency in the relevant wavelength range, are capable of detecting single photons while providing excellent spatial resolution and very low background noise. These are key requirements to allow pattern-recognition algorithms to reconstruct Cherenkov rings even in the high-occupancy region.
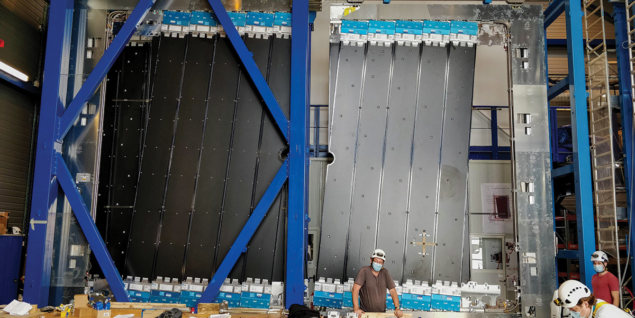
More than 3000 MaPMT units, for a total of 196,608 channels, are needed to fully instrument both upgraded RICH detectors. The already large active area (83%) of the devices has been maximised by arranging the units in a compact and modular “elementary cell” containing a custom-developed, radiation-hard eight-channel ASIC called the Claro chip, which is able to digitise the MaPMT signal at a rate of 40 MHz. The readout is controlled by FPGAs connected to around 170 channels each. The prompt nature of Cherenkov radiation combined with the performance of the new opto-electronics chain will allow the RICH systems to operate within the LHC’s 25 ns time window, dictated by the bunch-crossing period, while applying a time-gate of less than 6 ns to provide background rejection.
To keep the new RICHes as compact as possible, the hosting mechanics has been designed to provide both structural support and active cooling. Recent manufacturing techniques have enabled us to drill two 6 mm-diameter ducts over a length of 1.5 m into the spine of the support, through which a coolant (the more environmentally friendly Novec649, as in the SciFi tracker) is circulated. Each element of the opto-electronics chain has been produced and fully validated within a dedicated quality-assurance programme, allowing the position of the photon detectors and their operating conditions to be fine-tuned across the RICH detectors. In February, the first photon-detector plane of RICH2 (see “RICH2 to go” image) became the first active element of the LHCb upgrade to be installed in the cavern. The two planes of RICH2, located at the sides of the beampipe, were commissioned in early summer and will see first Cherenkov light during an LHC beam test in October.
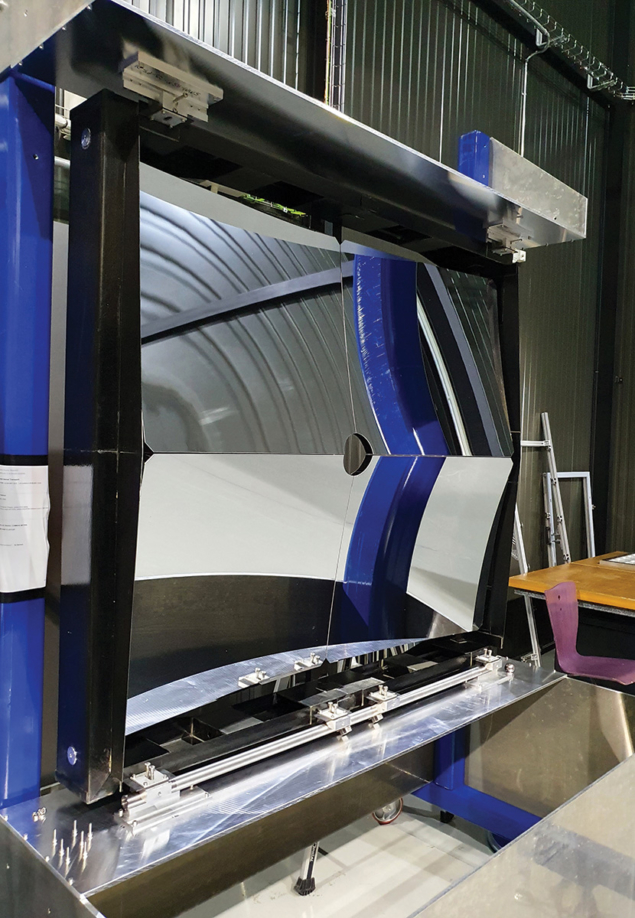
RICH1 presents an even bigger challenge. To reduce the number of photons in the hottest region, its optics have been redesigned to spread the Cherenkov rings over a larger surface. The spatial envelope of RICH1 is also constrained by its magnetic shield, demanding even more compact mechanics for the photon-detector planes. To accommodate the new design of RICH1, a new gas enclosure for the radiator is needed. A volume of 3.8 m3 of C4F10 is enclosed in an aluminium structure directly fastened to the VELO tank on one side and sealed with a low-mass window on the other, with particular effort placed on building a leak-less system to limit potential environmental impact. Installing these fragile components in a very limited space has been a delicate process, and the last element to complete the gas-enclosure sealing was installed at the beginning of June.
The optical system is the final element of the RICH1 mechanics. The ~2 m2 spherical mirrors placed inside the gas enclosure are made of carbon fibre composite to limit the material budget (see “Cherenkov curves” image), while the two 1.3 m2 planes of flat mirrors are made of borosilicate glass for high optical quality. All the mirror segments are individually coated, glued on supports and finally aligned before installation in the detector. The full RICH1 installation is expected to be completed in the autumn, followed by the challenging commissioning phase to tune the operating parameters to be ready for Run 3.
Surpassing expectations
In its first 10 years of operations, the LHCb experiment has already surpassed expectations. It has enabled physicists to make numerous important measurements in the heavy-flavour sector, including the first observation of the rare decay B0s → µ+µ–, precise measurements of quark-mixing parameters, the discovery of CP violation in the charm sector, and the observation of more than 50 new hadrons including tetraquark and pentaquark states. However, many crucial measurements are currently statistically limited, including those underpinning the so-called flavour anomalies (see Bs decays remain anomalous). Together with the tracker, trigger and other upgrades taking place during LS2, the new SciFi and revamped RICH detectors will put LHCb in prime position to explore these and other searches for new physics for the next 10 years and beyond.