VENUS, the latest superconducting ECR ion source, is blazing the trail for the next generation of heavy-ion accelerators, as Daniela Leitner of LBNL explains.
A key technology for the next generation of heavy-ion accelerators will be a powerful, high-charge-state heavy-ion injector that provides an ion-beam intensity an order of magnitude higher than is currently achievable. In addition, future facilities – such as the proposed Rare Isotope Accelerator (RIA) in the US, the Radioactive Ion Beam Factory at RIKEN in Japan, and the project to upgrade the facility at the Gesellschaft für Schwerionenforschung (GSI) in Germany – will demand a high flexibility in the species of ions available for experiments that may last several weeks. High-performance electron cyclotron resonance (ECR) ion sources routinely produce beams of ions ranging from hydrogen to uranium, thereby providing the necessary reliability and flexibility. However, to meet the requirements for high currents, a new generation of ECR ion source will be needed.

The Versatile ECR Ion Source for Nuclear Science (VENUS), designed and built at the Lawrence Berkeley National Laboratory (LBNL), is the most advanced superconducting ECR ion source and the first “next-generation” source in operation (figure 1). It is the first fully superconducting ECR ion source that reaches magnetic-confinement fields sufficient for optimum operation at 28 GHz. Recently the project passed a major milestone with the successful coupling of 28 GHz microwaves into the plasma of the ion source. Preliminary tests at this frequency have already resulted in record intensities for beams of medium and highly charged ions. The results indicate for the first time that the high demands of the next generation of heavy-ion accelerators can be met.
The development of ECR ion sources has its roots in fusion plasma research in the late 1960s. The principle is to use magnetic confinement and ECR heating to produce a plasma made up of energetic electrons and relatively cold ions. Figure 2 shows the main ingredients of an ECR ion source: magnets for plasma confinement, microwaves for ECR heating, and gas to create and sustain the plasma. For high-charge-state sources the magnetic confinement consists of an axial magnetic-mirror field superimposed by a radially increasing sextupole (also called hexapole) or other multipole magnetic field. The combination of the axial mirror field and the radial multipole field produces a “minimum-B” configuration, in which the magnetic field is at a minimum at the centre of the device and increases in every direction away from the centre, providing stable plasma confinement.
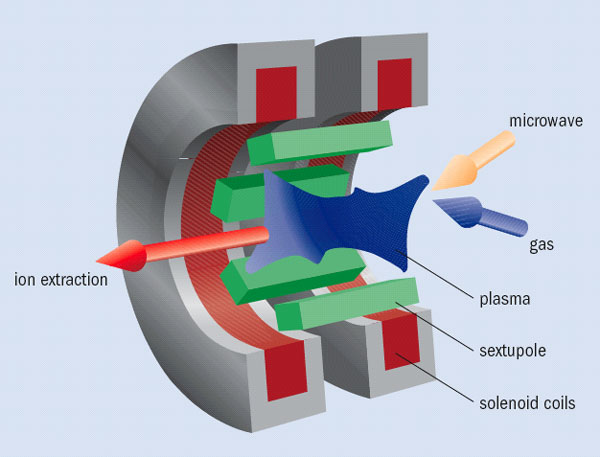
The electrons, which are heated resonantly by microwaves, produce the high-charge-state ions primarily by sequential impact ionization of atoms and ions in the plasma. The ions and electrons must be confined for long enough for this sequential ionization to take place. In a typical ECR ion source, ions need to be confined for about 10-2 s to produce high-charge-state ions. The ionization rate depends on the plasma density, which typically ranges from about 1011 cm-3 for low-frequency sources to more than 1012 cm-3 for the highest-frequency sources. Charge exchange with neutral atoms must be minimized, so operating pressures are typically 10-6 mbar or less. The plasma chamber is biased positively so that the ions can be extracted from the plasma and accelerated into the beam-transport system.
The first sources using ECR heating to produce multiply-charged ions were reported in 1972 in France by Richard Geller. Since then the development and refinement of ECR ion sources have improved performance dramatically. For example, in 1980 the Micromafios source at the Centre d’Etudes Nucléaires de Grenoble produced 20 eμA of Ar8+ and 10 eμA of Ar9+. Later the 18 GHz source at RIKEN produced 2000 eμA of Ar8+ and 1000 eμA of Ar9+ in 2003.
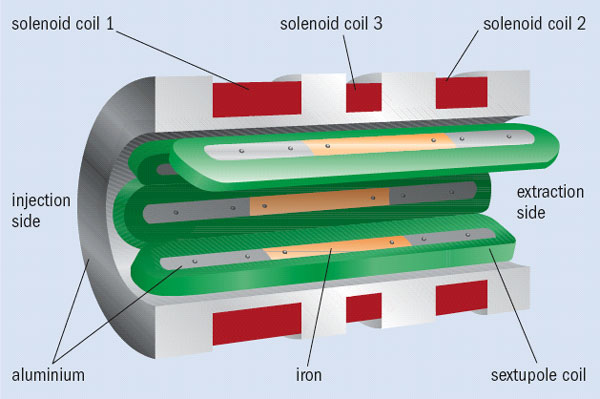
The main drivers for improving the performance of ECR ion sources were formulated in Geller’s famous ECR scaling laws, which predicted that higher magnetic fields and higher frequencies would increase both plasma density and ion-confinement times, which would improve performance. Following these guidelines and using other experimental data, the ambitious design for the VENUS ECR ion source was developed with magnetic-confinement fields much greater than those of previous sources. The strong forces between the superconducting sextupole and the solenoid coils were the main technical challenges of building such a source. Indeed, VENUS was the first source to build such a strong confinement structure, and it holds the world record for the highest ECR confinement field ever achieved, with an axial field of 4 T at injection, 3 T at extraction and a radial field at the plasma chamber wall of 2.4 T.
The technology that made this high field-strength possible was the careful design of the magnet-clamping structure, utilizing bladders filled with liquid metal and a split-pole structure made from iron and aluminium for the sextupole coils. The iron increases the radial field-strength by about 10%, and the aluminium pieces were used to match the thermal expansion of the superconducting wire and the pole. Figure 3 shows the conceptual design of the magnet structure.
Originally VENUS was designed to produce high-current, high-charge-state ions for the 88 inch cyclotron at LBNL, but it has evolved to serve also as the prototype injector ion source for the driver linac of the proposed RIA facility. In the latter application VENUS has become a highly visible project. Similar injector sources are proposed or under construction in RIKEN, GSI and the Laboratori Nazionali del Sud, Catania, in Italy.
Testing, testing…
The operational experience with VENUS has been excellent in terms of stability, reproducibility and reliability during the commissioning period with power at 18 and 28 GHz. During initial operation at 28 GHz, record intensities of medium-charge-state beams such as 245 eμA of Bi29+, and high-charge-state beams such as 16 eμA of Bi41+, were extracted easily. The testing programme has initially focused on bismuth, since its mass is close to that of uranium, which will be the most challenging ion beam for RIA and also for the radioactive ion-beam factory in RIKEN. Bismuth is an ideal test beam since it is less reactive than uranium, not radioactive and evaporates at modest temperatures. However, the processes of extraction and ion-beam formation, as well as the transport characteristics, are very similar to those for uranium. Moreover, bismuth is also very similar to lead, so the results could also be of interest for a future intensity upgrade for the Large Hadron Collider at CERN.
The preliminary performance data measured at 28 GHz in 2004 with VENUS confirmed the scaling laws for intensity, and were the first evidence that meeting the high-intensity requirements is feasible. Nevertheless, these high-intensity beams present a new challenge for the beam-transport lines of ECR ion sources, which are traditionally designed for low-current ion beams. In addition, the high magnetic field at the extraction region greatly affects ion-beam formation and quality (i.e. emittance). This could appear to limit a further increase in the confinement fields and heating frequencies. However, experiments have found that higher-charge-state beams have much higher beam quality than lower-charge-state beams.
This can be explained by a model where the high-charge-state ions are extracted closer to the magnetic-field axis than the low-charge-state ions, leading to less angular momentum and a smaller transverse beam emittance. VENUS’s widely variable magnetic field at extraction will enable us to explore this model experimentally. Later this year the VENUS source will be tested with uranium ions – one of the key ion beams for RIA and for the RIKEN radioactive ion-beam factory – which will be a major milestone for the project.