Innovation at the next-generation B factory to be built in Italy.
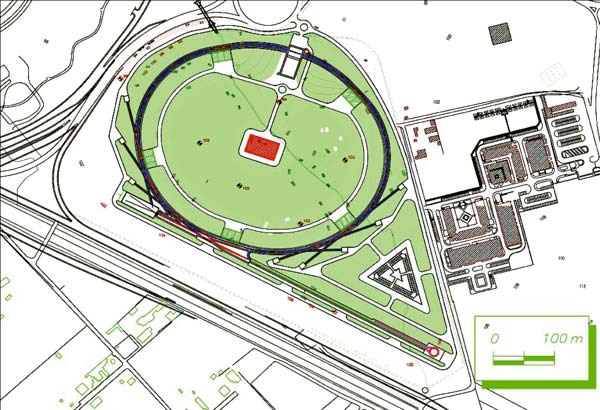
In 2010 the Italian government gave the green light for SuperB – a next-generation B factory based on an asymmetric electron–positron (e+e–) collider, which is to be constructed on the Tor Vergata campus of Rome University (figure 1). The intention is to deliver a peak luminosity of 1036 cm–2 s–1 to allow the indirect exploration of new effects in the physics of heavy quarks and flavours through studies of large samples of B, D and τ decays. Building on the wealth of results produced by the previous two B Factories, PEP-II and KEKB, and their associated detectors, BaBar and Belle, SuperB will produce an unprecedented amount of data and make accessible a range of new investigations.
The SuperB concept represents a real breakthrough in collider design. The low-emittance ring has its roots in R&D for the International Linear Collider (ILC) and could be used as a system-test for the design of the ILC damping ring. The invention of the crab-waist final focus could also have an impact on the current generation of circular colliders.
The SuperB e+e– collider will have two rings with a 1.25 km circumference, one for electrons at 4.18 GeV and one for positrons at 6.7 GeV. There will be one interaction point (IP) where the beams will be squeezed down to a vertical size of only 36 nm rms. The design results from a combination of knowledge acquired at the previous B factories as well as the concepts developed for linear colliders.
The innovative crab-waist principle, which has been successfully tested at Frascati’s Φ factory – the DAΦNE e+e– collider – will allow SuperB to overcome some of the requirements that have proved problematic in previous e+e– collider designs, such as high beam currents and short bunches. While SuperB will have beam currents and bunch lengths similar to those of its predecessors, the use of smaller emittances and the crab-waist scheme for the collision region should produce a leap in luminosity from some 1034 cm–2 s–1 to an unprecedented level of 1036 cm–2 s–1, without increasing the background levels in the experiments or the machine’s power consumption.
High luminosity in particle colliders not only depends on high beam-intensity, it also requires a small horizontal beam size and horizontal emittance (a measure of the beam phase space) and a very small value for the vertical β function at the IP, β*y. (The β function in effect gives the envelope of the possible particle trajectories and has a parabolic behaviour around the IP.) However, β*y cannot be made much smaller than the bunch length without running into trouble with the “hourglass” effect, in which particles in the bunch-tails experience a much higher β*y and a loss in luminosity.
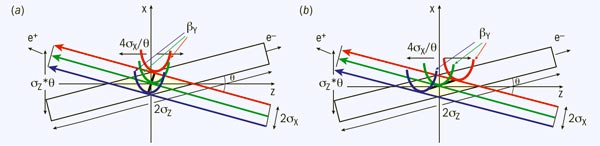
Unfortunately it is difficult to shorten the bunch length in a high-current ring without exciting instabilities and therefore paying in radio-frequency voltage. One way to overcome this is to make the beam crossing-angle relatively large and the horizontal beam size small, so that the region where the two colliding beams overlap is much smaller than the bunch length. In addition, in the crab-waist scheme, two sextupoles at suitable phase-advances from the IP are used to rotate the waist in the β function of one beam such that its minimum value is aligned along the trajectory of the other beam, so maximizing the number of collisions occurring at the minimum β (figure 2). This technique can substantially increase luminosity without having to decrease the bunch length. A crab-waist scheme was tested at the DAΦNE in 2008 allowing a peak luminosity three times higher than the previous record for similar currents in the two rings.
The combination of large crossing-angle and small beam sizes, emittances and beam angular divergences at the IP in the SuperB design will also be effective in decreasing the backgrounds present at the IP with respect to the previous B factories. A limited beam current also contributes to keeping these levels very low at SuperB. However, luminosity-related backgrounds are still relevant and impose serious shielding requirements.
The high luminosity of SuperB, representing an increase of nearly two orders of magnitude over the current generation of B factories, will allow exploration of the contributions of physics beyond the Standard Model to the decays of heavy quarks and heavy leptons. Indeed, new physics can affect rare B-decay modes through observables such as branching fractions, CP-violating asymmetries and kinematic distributions. These decays do not typically occur at tree level, so their rates are strongly suppressed in the Standard Model. Substantial variations in the rates and/or in angular distributions of final-state particles could result from the presence of new heavy particles in loop diagrams, providing clear evidence of new physics. Moreover, because the pattern of observable effects is highly model-dependent, measurements of several rare decay modes can provide information regarding the source of the new physics.
The SuperB data sample will contain unprecedented numbers of charm-quark and τ-lepton decays. Such data are of great interest, both in a capacity to improve the precision of existing measurements and in sensitivity to new physics. This interest extends beyond weak decays; the detailed exploration of new charmonium states is also an important objective. Limits on rare τ decays, particularly lepton-flavour-violating decays, already provide important constraints on models of new physics and SuperB may have the sensitivity to observe such decays. The accelerator design will allow for longitudinal polarization of the electron beam, making possible uniquely sensitive searches for a τ electric dipole moment, as well as for CP-violating τ decays.
Studies of CP-violating asymmetries are among the primary goals of SuperB. In addition to known sources of CP, new CP-violating phases arise naturally in many extensions of the Standard Model. These extra phases produce measurable effects in the weak decays of heavy-flavour particles. The detailed pattern of these effects, as well as of rare-decay branching fractions and kinematic distributions, will be made accessible by SuperB’s high luminosity. Such studies will provide unique constraints in, for example, ascertaining the type of supersymmetry breaking or the kind of extra-dimensional model behind the new phenomena. A natural consequence of such detailed studies will be an improved knowledge of the unitarity triangle to the limit allowed by theoretical uncertainties.
In addition to pursuing important research in fundamental physics, SuperB is also taking up the challenge to combine it with a rich programme of applied physics: the synchrotron light emitted by the machine will have a high brightness and will be suitable for studies in life sciences and material science. Current proposals include: the creation and exploitation of beamlines for laser ablation on biomaterials (a technique that, by modifying the surface of the material with a laser, allows the creation of patterns of biological systems); femtochemistry studies (a field that includes the structural study of small numbers of molecules); and the development of new phase-contrast imaging techniques to improve the reconstruction of morphological information related to tissues and organs.
The construction of SuperB, which is funded by the Italian government and supported by a large international collaboration that includes scientists from Europe, the US and Canada, is planned to take about six years. The newly established “Nicola Cabibbo Laboratory” Consortium will provide the necessary infrastructure for the exploitation of the new accelerator. In November, the Consortium appointed Roberto Petronzio as director with an initial three-year mandate. The machine will reuse several components from PEP-II, such as the magnets, the magnet power-supplies, the RF system, the digital feedback-system and many vacuum components. This will reduce the cost and engineering effort needed to bring the project to fruition.
The exciting physics programme foreseen for SuperB can only be accomplished with a large sample of heavy-quark and heavy-lepton decays produced in the clean environment of an e+e– collider. The programme is complementary to that of an experiment such as LHCb at a hadron collider. Indeed, a “super” flavour factory such as SuperB will, perforce, be a partner together with experiments at the LHC, and eventually at an ILC, in ascertaining exactly what kind of new physics nature has in store.