An interview with the 2005 Nobel laureate explores his links with particle physics and CERN.
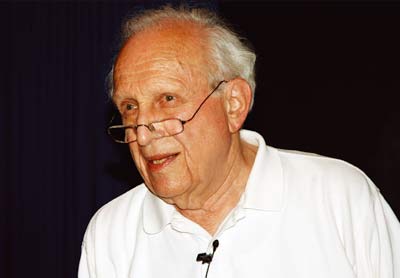
When Roy Glauber was a 12-year-old schoolboy he discovered the beauty of making optical instruments, from polarizers to telescopes. His mathematical skills stem from those early school days, when a teacher encouraged him to begin studying calculus on his own. When he progressed to Harvard in 1941 he was already a couple of years ahead and had absorbed a fair fraction of graduate-level studies by 1943, when he was recruited into the Manhattan Project at the age of 18. It was then that the erstwhile experimentalist began the transition to theoretician. Finding the experimental work rather less demanding than theory – “It seemed to depend on how to keep a good vacuum in a counter,” he recalls, “and I didn’t think I would do it any better” – he asked to join the Theory Division and was set to work on solving neutron-diffusion problems.
Following the war, Glauber gained his BSc and PhD from Harvard and after apprenticeships with Robert Oppenheimer in Princeton and Wolfgang Pauli in Zurich, he stood in for Richard Feynman for a year at Caltech and then settled back at Harvard in 1952. By this time, he says, “all of the interest was in nuclear physics studied through scattering experiments”. With increasing energies becoming available at particle accelerators, the wavelength associated with the incident particles was decreasing to nuclear dimensions and below. Viki Weisskopf and colleagues had already developed the cloudy crystal-ball model of the nucleus, which successfully described averaged neutron cross-sections, and Glauber believed that the idea could be extended. “I had this conviction that it ought to be possible to represent the nucleus as a semi-translucent ball, from 20 MeV up,” he recalls. However, what the optical models lacked, in Glauber’s view, “was a proper quantitative derivation based on the scattering parameters of individual nucleons”.
Inspired by work on electron diffraction by molecules that he had pursued at Caltech, Glauber began to think about how to apply optical Fraunhofer-diffraction theory to higher-energy nuclear collisions – in a sense, bringing about a fusion of two of his interests. At higher energies, he argued, individual collisions could be treated diffractively and allow nuclear calculations to be based on the familiar ground of optical-diffraction theory.
The result was a generalized nuclear diffraction theory, in which he introduced charges and internal co-ordinates that did not exist in the optical case, such as spin and isospin, and dealt with scattering from nuclei that contained many nucleons by treating arbitrary numbers of successive collisions. The key was to consider energy transfers that were small compared with the incident energy. This was a reasonable assumption at higher energies and it led to a useful approximation method that provided a mathematical development of the original optical model, and allowed treatment of the preponderance of inelastic transitions.

The theory turned out to work quite well for proton–deuteron and proton–helium collisions in experiments at the Cosmotron at Brookhaven. “You could see single and double scattering in the deuteron and helium,” he explains, “and shadowing” – where target nucleons lie in the shadow of others. However, at the time there were no studies of heavier nuclei.
Glauber made the first of many visits to CERN in 1964 and arrived for a six-month sabbatical in February 1967. “It was a most dramatic time for me,” he recalls. The group led by Giuseppe Cocconi had begun measurements of proton scattering from nuclear targets using the first extracted-proton beam from the PS. They made a series of measurements at 19.3 GeV/c but with the resolution of the spectrometer limited to 50 MeV, they could not separate elastic from inelastic scattering. Glauber realized that, extended to inelastic scattering, the theory would cover essentially all nuclear excitations in which there was no production of new particles. Together, the calculated elastic and inelastic cross-sections agreed exactly with what Cocconi’s group was measuring. Glauber presented the results of his work with Giorgio Matthiae of Cocconi’s group at a meeting in Rehovot in the spring of 1967. “We were doing quantitative high-energy physics for a change,” he says.
The work at CERN with Cocconi’s group left a big impression on Glauber: “It was something wonderful and inspiring.” He became “hooked on CERN”, returning many times for summers and sabbaticals, working on models for elastic scattering for experiments at the ISR and for UA4 on the SPS proton–antiproton collider. However, by the 1990s – the era of the Large Electron–Positron (LEP) collider – his visits became less frequent. “I found I had nothing new to say about LEP cross-sections,” he admits.
Today there is renewed interest in Glauber’s work, in particular among physicists involved with heavy-ion collisions. His early calculations of multiple diffraction laid the foundations for ideas that are central (in more ways than one) to studies in which nuclei collide at very high energies. The basic formalism of overlapping nucleons can be used to calculate the “centrality” of a collision – in other words, how head-on it is. However, other work in the field of optical theory also finds relevance in the unusual environment of heavy-ion collisions – in this case Glauber’s work on a quantum theory of optical coherence, which led to his share of the Nobel prize in 2005.
This work again dates back to the late 1950s and the discovery by Robert Hanbury-Brown and Richard Twiss of correlations in the intensities measured by two separated photon detectors observing the same light source. Their ultimate aim had been to extend their pioneering work on intensity interferometry at radio wavelengths to the optical region, so as to measure the angular sizes of stars – which they went on to do for Sirius and others. However, they first set up an experiment in the laboratory to reassure themselves that the technique would work at optical wavelengths. The result was surprising: light quanta have a significant tendency to arrive in pairs, with a coincidence rate that approaches twice that of the random background level. Extending the idea led to predictions that a laser source, with its narrow bandwidth, should show a large correlation effect. Glauber was sceptical, so he embarked on a proper quantum-theoretical treatment of the statistics of photon detection.
“Correlated pairs are characteristic of unco-ordinated chaotic emission from lots of sources,” he explains, “where the statistics are Gaussian. This is not a characteristic of light from a laser where all of the atoms know quite well what the other atoms are doing.” He realized correctly that this co-ordination means that there should be no Hanbury-Brown–Twiss correlation for a laser source and he went on to lay down the theoretical ground work for the field of quantum optics – the work that led to the Nobel prize.
There are similarities between the statistics in the detection of photons (bosons) and those of the detection of pions (also bosons) in heavy-ion collisions. The energetic collision should be like a thermal light source, with correlated pion emission akin to the Hanbury-Brown–Twiss correlations allowing the possibility of measuring the size of the source, as in the astronomical studies. Experiments do find such an effect but they do not see the full factor of two above the random background and the reason is yet to be properly understood. While the width of the measured peak may relate to the radius of the source, “we don’t have a theory of the radiation process that explains fully the correlation”, says Glauber, “no real quantitative explanation. Perhaps other things are upsetting the correlations.”
The LHC will explore further the realm of heavy-ion collisions and push on with measurements of the proton–proton total cross-section, a focus of the TOTEM experiment. While these links remain between his work and CERN, Glauber observes that the laboratory has changed a great deal since his first visits, but he is still “very devoted to the place as an ideal”. What then, does he hope in general for the LHC? “Pray to find a surprise,” he says. “It may be difficult to design an experiment to detect what you least expect, but we really need some surprises.”
• For Roy Glauber’s colloquium at CERN on 6 August, see http://indico.cern.ch/conferenceDisplay.py?confId=62811.