TOTEM prepares innovative detectors to study collisions at the LHC.
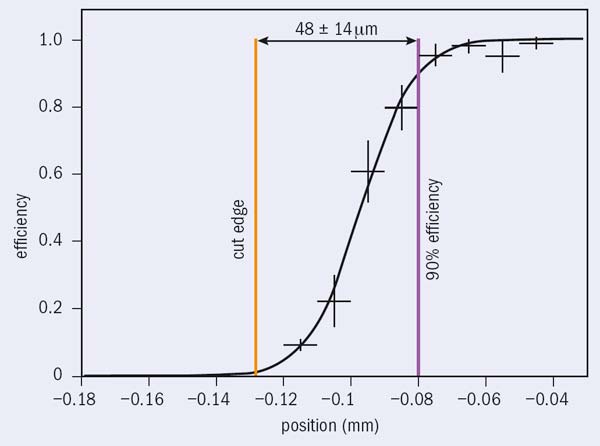
While most of the LHC experiments are on a grand scale, the subdetectors for TOTEM, which stands for TOTal cross-section, Elastic scattering and diffraction dissociation Measurement at the LHC, are not longer than 3 m, although they extend over more than 440 m. Despite reduced dimensions, TOTEM’s potential resides in making some unique observations. In addition to the precise measurement of the proton–proton interaction cross-section, TOTEM’s physics programme will focus on the in-depth study of the proton’s structure by looking at elastic scattering over a large range of momentum transfer. Many details of the processes that are closely linked to proton structure and low-energy QCD remain poorly understood, so TOTEM will investigate a comprehensive menu of diffractive processes – the latter partly in co-operation with the CMS experiment, which is located at the same interaction point on the LHC.
The measurement of the total proton–proton interaction cross-section with a luminosity-independent method requires a detailed study of elastic scattering down to small values of the squared four-momentum transfer, together with the measurement of the total inelastic rate. Early measurements at CERN’s Intersecting Storage Rings (ISR), which were confirmed at CERN’s SppS collider and at the Tevatron at Fermilab, revealed that the proton–proton interaction probability increases with collider energy. However, the nature of the correct growth with energy remains a delicate and unresolved issue. A precise measurement of the total cross-section at the world’s highest-energy collider should discriminate between the different theoretical models that describe the energy dependence. The value of the total cross-section at LHC energies is also important for the interpretation of cosmic-ray air showers. All of the LHC experiments will use TOTEM’s measurement to calibrate their luminosity monitors, in order to calculate the probability of measuring rare events.
Sophisticated detectors
The study of physics processes in the region close to the particle beam, which is complementary to the programmes of the LHC general-purpose experiments, requires appropriate detectors. In the case of elastic and (most) diffractive events, intact protons in the final state need to be detected at a small angle relative to the beam line, therefore special proton detectors must be inserted into the vacuum beam pipe of the LHC. The TOTEM Collaboration had to invest heavily in the design of sophisticated detectors characterised by a high acceptance for particles produced in the busy region close to the beam pipes. All of the three subdetectors – Roman Pots and two particle telescopes, T1 and T2 – will detect charged particles emitted by the proton–proton collisions at interaction point 5 (IP5) on the LHC and will have trigger capabilities to allow an online selection of specific events.

The Roman Pots are special movable devices that are inserted directly into the beam pipe by bellows, which are compressed as the pots are pushed towards the beams circulating inside the vacuum pipe. They are called “Roman” because they were first used by a group of Italian physicists from Rome, in the early 1970s, to study similar physics at the Intersecting Storage Rings, the world’s first high-energy proton–proton collider. They are known as “Pots” because the vessels that house the delicate detectors, which can localize the trajectory of protons passing within 1 mm of the beam (with a precision of around 20 μm), are shaped like a vase.
In the TOTEM experiment, there are four Roman Pot stations, each composed of two units, separated by a distance of a few metres. Each unit consists of two pots in the vertical plane, which approach the beam from above and below, and one pot that moves horizontally. They are placed on both sides of the interaction point, at distances of 147 m and 220 m.
The proton detectors in the Roman Pots are silicon devices designed by Vladimir Eremin, Nikolai Egorov and Gennaro Ruggiero with the specific objective of reducing the insensitive area at the edge facing the beam to only 50 μm. This can be compared with a dead area typically more than 10 times larger for silicon detectors currently used elsewhere. High efficiency up to the physical border of the detector is an essential feature to maximize the experiment’s acceptance for protons scattered elastically or diffractively at polar angles down to a few microradians at the interaction point. Radiation-hardness studies indicate that this edgeless detector remains fully efficient up to a fluence of about 1.5 × 1014 protons/cm2.

Image credit: TOTEM.
The inelastic rate is measured by the telescopes T1 and T2. These are two charged-particle trackers situated close to the beam pipes in the CMS cavern at distances of about 10.5 m and 13.5 m on either side of the interaction point; indeed, T1 is within the CMS end-cap. By providing a full azimuthal coverage around the beam line, these telescopes will be able to reconstruct the tracks of charged particles coming from the proton–proton collisions and so allow the determination of the primary interaction vertex.
Each T1 tracker is made up of five subdetector planes perpendicular to the beam line. Each plane consists of six cathode-strip chambers (CSCs) – multiwire proportional chambers filled with a gas mixture, with cathode layers segmented into parallel strips. The advantages of this kind of detector are that it utilizes a well proven technology, provides a simultaneous measurement of three spatial co-ordinates (one from the anode wire plane and two from the cathode-strip planes) and uses a safe gas mixture (Ar/CO2/CF4). As T1 is installed in a high-radiation environment, the chambers have been tested in the gamma-irradiation facility at CERN. They have shown stable performances at doses several times higher than those expected for the design running conditions and exposure time. Tests with cosmic rays and muon beams have shown performances as expected.
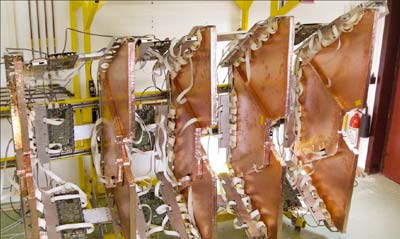
Image credit: TOTEM.
The T2 tracking chambers are based on the gas electron multiplier (GEM) technology, invented by Fabio Sauli and Leszek Ropelewski at CERN, which combines a good spatial resolution with a high rate capability and a good resistance to radiation. In each T2 arm, 20 semi-circular GEM planes, with overlapping regions, are interleaved on both sides of the beam vacuum chamber to form 10 detector planes with full azimuthal coverage. In GEM detectors, in contrast to CSCs, the signal is collected on thin polyimide foils covered by a thin layer of copper on both sides. These foils, densely pierced and contained between two electrodes, are able to achieve high amplification and performance. GEM technology was chosen for T2 for the radiation hardness of the chambers and the flexibility of the read-out geometry. The read-out plane in the T2 chambers has been designed with strips that give a good resolution on the pseudo-rapidity co-ordinate, while pads give the phi co-ordinate for tracking and trigger purposes. Assembled “quarters” were tested with cosmic rays before the installation at IP5 and precommisioning tests have shown a good efficiency and resolution, matching the expected values.
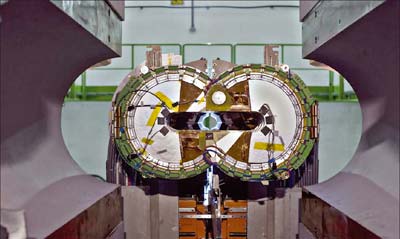
The read-out of all TOTEM sub-systems is based on the custom-developed digital Very Forward ATLAS–TOTEM (VFAT) chip, which also contains trigger capability. The data acquisition (DAQ) system is designed to be compatible with the CMS DAQ to make common data-taking possible at a later stage.

The collaboration has recently completed the installation of the Roman Pot stations at 220 m and the subdetector T2. T1 is going to be installed in autumn. In the future two more Roman Pot stations will be put in place at 147 m. The first measurements of the LHC luminosity and individual cross-sections will be performed by TOTEM as soon as the LHC collider becomes operational. The collaboration is looking forward to having adequate data to carry out their first new physics analyses and to having results to announce in 2010.
• The TOTEM Collaboration has about 100 members from 10 institutions in seven countries. Karsten Eggert from CERN is the spokesperson; Angelo Scribano, from the University of Siena and INFN Pisa, is the chair of the Collaboration Board; and Ernst Rademacher from CERN is the technical co-ordinator.