Experiments at Cornell are breaking records for accelerating gradients and Q values in superconducting radio-frequency cavities, reports Hasan Padamsee.
Improvements in our understanding of the mechanisms that limit accelerating gradients or electric fields, together with technological advances from worldwide R&D, have steadily increased the performance of superconducting cavities over the past decade.
The TESLA collaboration is now achieving accelerating gradients of 35 MV/m in 1 m-long superconducting structures suitable for the proposed 500 GeV International Linear Collider. The best single-cell cavities at many laboratories reach 40-42 MV/m. At these gradients, energy losses from the superconducting microwave cavity resonators are still miniscule, with “intrinsic Q” values exceeding 1010, i.e. it takes 1010 oscillations for the stored energy in the resonator to die out. If Galileo’s original pendulum oscillator had possessed a similar Q value, it would still be oscillating now, 400 years later.

One goal of future R&D programmes is to push accelerating gradients and Q values even higher either to reach tera-electron-volt energies or to save on costs. However, above 40 MV/m the magnetic field at the surface of the resonator approaches the fundamental limit where superconductivity breaks down. One way to circumvent this limit is to modify the shape of the cavity to reduce the ratio between the peak magnetic field and the accelerating field.
About two years ago, Valery Shemelin, Rongli Geng and Hasan Padamsee at the Cornell University Laboratory for Elementary- Particle Physics (LEPP) introduced a “re-entrant” shape, which lowers the surface magnetic field by 10%. Figure 1 compares the re-entrant cavity shape and the shape of the TESLA cavity. The downside of the new shape is the higher accompanying surface electric fields, which enhance “field emission” of electrons from the regions of high electric field. Field emission does not present a “brick wall” limit, however, because techniques such as high-pressure rinsing with high-purity water at pressures of about 100 bar eliminate the microparticle contaminants that cause field emission.
Another important aspect of cavity shape is beam aperture. When a bunch of charge passes through an accelerating cavity it leaves behind a wakefield, which disrupts oncoming bunches. Smaller apertures produce stronger wakefields. The re-entrant shape has the same aperture as the TESLA shape; nevertheless, reducing the aperture, say from 70 to 60 mm, would yield higher accelerating gradients because it would allow a surface magnetic field 16% lower. Further studies are in progress to evaluate the trade-off between higher wakefields and higher potential accelerating gradients.
New ideas are usually proved in single-cell cavities before the technical challenges of multi-cell accelerating units are addressed. The first 70 mm-aperture re-entrant single-cell cavity fabricated at Cornell reached a world record accelerating field of 46 MV/m at a Q value of 1010, and 47 MV/m in the pulsed mode, which is suitable for a linear collider. Figure 2 shows how Q varies with accelerating field for the cavity. To reach these record performance levels, the cavity was made from high-purity, high-thermal-conductivity niobium (with residual resistivity ratio of 500) to avoid thermal breakdown of the superconductivity. Electropolishing provided an ultra-smooth surface.
High-pressure rinsing at 100 bar thoroughly scrubbed the surface free of the microparticles that cause field emission. Final assembly took place in a Class-100 clean-room environment. All these are now standard techniques for the best superconducting cavity preparation. In addition, baking at 100 °C for 50 h promoted a redistribution of the oxygen in the radio-frequency (RF) layer, which is known to avoid premature RF losses.
Record operating Qs
When operating an accelerating cavity with beam, another important Q value is the “operating” or “loaded” Q. This is determined by the power lost to the beam, whereas the “intrinsic Q” is determined by the ohmic power loss in the cavity walls. Intrinsic Q values are 1010 or higher as discussed above. For applications with minimal beam loading, the closer loaded Q is to intrinsic Q, the smaller the overall RF power investment and operating costs.
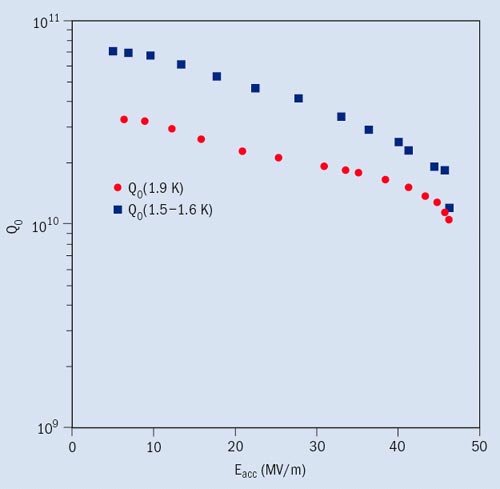
The state of the art for structures designed to accelerate velocity-of-light particles is operation at a loaded Q of 2 x 107. Higher loaded Qs are extremely challenging because the resulting bandwidth of the cavity resonance is only a few hertz (out of a typical 1.5 GHz), making the field in the cavity extremely sensitive to any perturbation of the resonance frequency due to microphonics or detuning of Lorentz forces. However, Qs above 108 are highly desirable for future applications, in particular for energy-recovery linacs (ERLs) for future high-flux, high-brilliance light sources. These are being pursued by many laboratories around the world, including Cornell. No control system has ever met the amplitude and phase-stability requirements of the RF field at a loaded Q of 108.
Building on techniques developed at DESY for the Tesla Test Facility, researchers at Cornell, under the direction of Matthias Liepe, have developed a new digital RF control system that provides great flexibility, high computational power and low latency for a wide range of control and data-acquisition applications. Recently Cornell tested this system in two extreme regimes of loaded Q. First, in the Cornell Electron Storage Ring (CESR), the system stabilized the vector-sum field of two of the ring’s superconducting 500 MHz cavities at a loaded Q of 2 x 105 with a beam current of several hundred milliamps. Several months of continuous operation proved the system’s high reliability and the field stability surpassed design requirements.
In a more crucial and demanding test, a team from Cornell and Jefferson Laboratory (JLab) connected the system to a cavity with a loaded Q greater than 108 at JLab’s infrared free-electron laser and tested it with beam in the energy-recovery mode, in which the effective beam current is practically zero. In continuous operation, excellent field stability – about 2 x 10-4 rms in relative amplitude and 0.03 degrees rms in phase – was achieved at a loaded Q of 1.4 x 108 in full energy-recovery mode. This sets a new record for loaded Q operation of linac cavities. At the highest loaded Q, less than 500 W of klystron power was required to operate the cavity at a field of 12 MV/m in energy-recovery mode with a beam current of 5 mA. At the more usual loaded Q of 2 x 107, about 2 kW is required.
The control system used includes digital and RF hardware developed in-house; very fast feedback and feed-forward controls; automatic start-up and trip recovery; continuous and pulsed-mode operation; fast quench-detection; and cavity-frequency control. The cavity-frequency control relied on a fast tuner based on a piezoelectric tuning element, which proved effective in keeping the cavity on resonance. As an added bonus, the ramp-up time to high gradients was less than 1 s, instead of the more usual minutes.