Intriguing hints of deviations from the Standard Model in the flavour sector point towards new physics accessible at a Future Circular Collider, write Andreas Crivellin and John Ellis.
Half a century after its construction, the Standard Model of particle physics (SM) still reigns supreme as the most accurate mathematical description of the visible matter in the universe and its interactions. It was placed upon its throne by the many precise measurements made at the Large Electron Positron collider (LEP), in particular, and its rule was confirmed by the discovery of the Higgs boson at the Large Hadron Collider (LHC). CERN’s LEP/LHC success story, in which a hadron collider provided direct evidence for a new particle (the Higgs boson) whose properties were already partially established at a lepton collider, can serve as a blueprint for physics discoveries at a proposed Future Circular Collider (FCC) operating at CERN after the end of the LHC.
Back in the late 1970s and early 1980s when the LEP/LHC programme was first proposed, the W and Z bosons mediating the weak interactions had not yet been observed, the top quark was considered a possible discovery, and the Higgs boson was regarded as a distant speculation. Precise studies of the W and Z, which were discovered in 1983 at the SPS proton–antiproton collider at CERN, were key items in LEP’s physics programme along with direct searches for the top quark, the Higgs boson and possible unknown particles. Even though the LEP experiments did not reveal any new particles beyond the W and Z, the unprecedented precision of its measurements revealed indirect effects (via quantum fluctuations) of the top and the Higgs, thereby providing indirect evidence for the SM mechanism of electroweak symmetry breaking. When the top quark was discovered at the Tevatron proton–antiproton collider at Fermilab in 1995, and the Higgs boson at the LHC in 2012, their masses were within the ranges indicated by precision measurements made at lepton colliders.
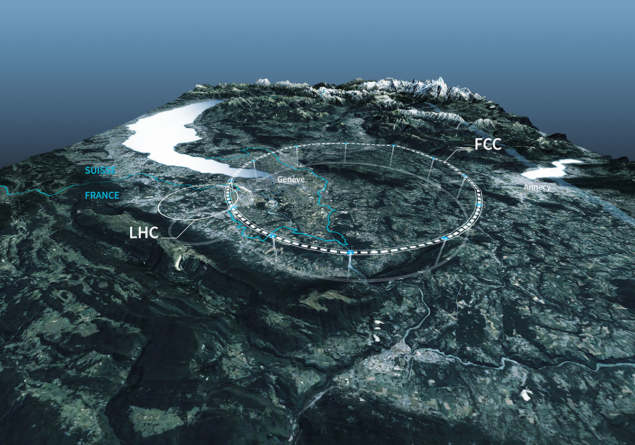
Nowadays, the hope is that the proposed FCC programme – comprising an electron–positron collider followed by a high-energy proton-proton collider in the same ~100 km tunnel – will repeat the LEP/LHC success story at an even higher level of precision and energy. The e+e– FCC stage would reproduce the entire LEP sample of Z bosons within a couple of minutes, yielding around 5 × 1012 Z bosons after four years of operation. In addition to allowing an incredibly accurate determination of the Z-boson’s properties, Z decays would also provide unprecedented samples of bottom quarks (1.5 × 1012) and tau leptons (3 × 1011). Potential increases in the FCC-ee centre-of-mass-energy would also produce unparalleled numbers of W+W– and top–antitop pairs, which are important for the global electroweak fit, close to their respective thresholds, as well as more Higgs bosons than promised by other proposed e+e– Higgs factories.
Probing beyond the Standard Model
Analyses of FCC-ee data, combined with results from previous experiments at the LHC and elsewhere, would not only push our understanding of the SM to the next level but would also provide powerful indirect probes of possible physics beyond the SM, with sensitivities to masses an order of magnitude greater than those of the LHC. A possible subsequent proton–proton FCC stage (FCC-hh) operating at a centre-of-mass energy of at least 100 TeV would then provide unequalled opportunities to discover this new physics directly, just as the LHC made possible the discovery of the Higgs boson following the indirect hints from high-precision LEP data. Whereas the combination of LEP and the LHC explore the TeV scale both indirectly and directly, the combination of FCC-ee and FCC-hh will carry the search for new physics to 30 TeV and beyond.
The e+e– stage of FCC would reproduce the entire LEP sample of Z bosons within a couple of minutes
However, for this dream scenario to play out, at least one beyond-the-SM particle must exist within FCC’s discovery reach. While the existence of dark matter and neutrino masses already prove that the SM cannot be complete (and there is no shortage of theoretical ideas as to what extensions of the SM could account for them), these observations can be explained by new particles within a very wide mass range – possibly well beyond the reach of FCC-hh. Fortunately, intriguing hints for new physics in the flavour sector have accumulated in recent years that point towards beyond-the-SM physics that should be accessible to FCC.
B-decay anomalies
Within the SM, the charged leptons – electrons, muons and taus – all have very similar properties. They interact with the photon as well as the W and Z bosons in the same way, and differ only in their masses, which in the SM are represented as Yukawa couplings to the Higgs boson. It is therefore said that the SM (approximately) respects lepton-flavour universality (LFU), despite the seemingly large differences in charged-lepton lifetimes originating from phase-space effects.
Flavour observables (i.e. processes resulting from rare transitions among the different generations of quarks and leptons), and observables measuring LFU in particular, are especially promising to test the SM because they are strongly suppressed in the SM and thus very sensitive to new physics. In recent years, a coherent pattern of anomalies, all pointing towards the violation of LFU, have emerged. Two classes of fundamental processes giving rise to decays of B mesons – b → sℓ+ℓ– and b → cτν – show deviations from the SM predictions.
In the flavour-changing neutral-current process b → sℓ+ℓ–, a heavy bottom quark undergoes a transition to a strange quark and a pair of oppositely-charged leptons, which could be either electrons or muons. The ratios RK = Br(B → Kμ+μ–)/Br(B → Ke+e–) and RK* = Br(B → K*μ+μ–)/Br(B → K*e+e–), measured most precisely by the LHCb collaboration, are particularly interesting because their SM predictions are very clean. Since the muon and electron masses are negligible compared to the B-meson mass, the ratio of muon to electron decays should be close to unity according to the SM. However, intriguingly, LHCb has observed values significantly lower than one, and recently reported first evidence for LFU violation in RK . These hints of new physics are supported by measurements of the angular observable P5′ in B0 → K*0μ+μ– decays and the rate of Bs → φμ+μ– decays. Importantly, all these observations can potentially be explained by the same new-physics interactions and are consistent with all other available measurements of processes involving b → sℓ+ℓ– transitions. In fact, global fits of all available b → sℓ+ℓ– data find a preference for new physics compared to the SM hypothesis which reeks of a possible discovery.

The second class of anomalies involves the charged-current process b → cτν, which is already mediated at tree level in the SM. The corresponding B-meson decays therefore have much higher probabilities to occur and thus larger branching ratios. However, the non-negligible tau mass leads to imperfect cancellations of the form factors in the ratio to electron or muon final states, and thus the resulting SM prediction is not as precise as those for RK and RK*. The most prominent examples of observables involving b → cτν transitions are the ratios RD = Br(B → Dτντ)/Br(B → Dℓνℓ) and RD* = Br(B → D*τ ντ)/Br(B → D*τνℓ). Here, the measurements of Belle, BaBar and LHCb consistently point above the SM predictions, resulting in a combined tension of 3σ. Importantly, as these processes happen quite frequently in the SM, a significant new-physics effect would be required to account for the corresponding anomaly.
With the FCC-ee capable of producing 1.5 × 1012 b quarks, clearly the b anomalies could be further verified within a short period of running, assuming that LHCb, Belle II and possibly other experiments do confirm them. The large data sample would also allow physicists to study complementary modes that bear upon LFU but are more difficult for LHCb to measure, such as other “R” measurements involving neutral kaons. These measurements would be invaluable for pinning down the mechanism responsible for any violation of lepton universality.
Other possible anomalies
The B anomalies are just one exciting avenue that a “Tera-Z factory” like FCC-ee could explore further. The anomalous magnetic moment of the muon, aμ, can also be viewed as an exciting hint for new physics in the lepton sector. Predicted by the Dirac equation to have a value exactly equal to two, the physical value of the magnetic moment of the muon is slightly higher due to fluctuations at the quantum level. The very high precision of both the calculation and measurement therefore make it a powerful observable with which to search for new physics. A tension between the measured and predicted value of aμ has persisted since Brookhaven published its final result in 2006, and was recently strengthened by the muon g-2 experiment at Fermilab, yielding an overall significance of 4.2σ when combined with the earlier Brookhaven data.
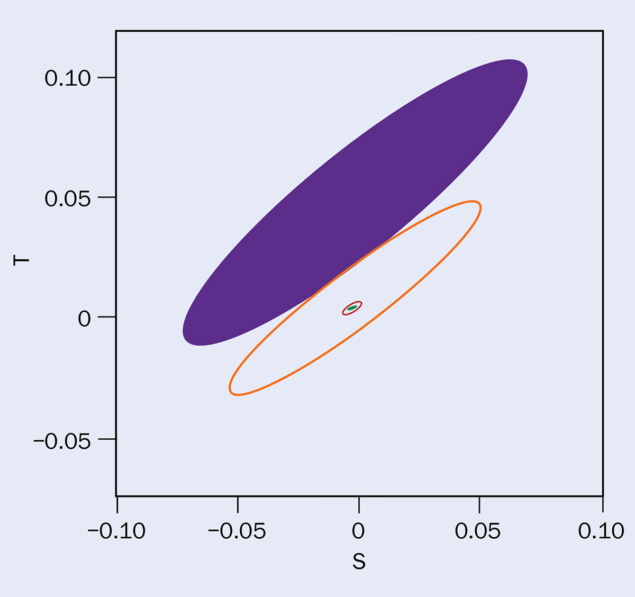
Various models have been proposed to explain the g-2 anomaly. They include leptoquarks (scalar or vector particles that carry colour and couple directly to a quark and a lepton that arise in models with extended gauge groups) and supersymmetry. Such leptoquarks could have masses anywhere between the lower LHC limit of 1.5 TeV and about 10 TeV, thus being within the reach of FCC-hh, whereas a supersymmetric explanation would require a couple of new particles with masses of a few hundred GeV, possibly even within reach of FCC-ee. Importantly, any explanation involving heavy new particles would also lead to effects in Z → μ+μ–, as both observables are sensitive to interactions with sizeable coupling strength to muons. FCC-ee’s large Z-boson sample could therefore reveal deviations from the SM predictions at the suggested level. Leptoquarks could also modify the SM prediction for H → μ+μ– decay, which will be measured very accurately at FCC-hh (see “Anomalous correlations” figure).
CKM under scrutiny
As the Cabibbo–Kobayashi–Maskawa (CKM) matrix, which describes flavour violation in the quark sector, is unitary, the sum of the squares of the elements in each row and in each column must add up to unity. This unitarity relation can be used to check the consistency of different determinations of CKM elements (within the SM) and thus also to search for new physics. Interestingly, a deficit in the first-row unitarity relation exists at the 3σ level. This can be traced back to the fact that the value of the element Vud, extracted from super-allowed beta decays, is not compatible with the value of Vus, determined from kaon and tau decays, given CKM unitarity. Interestingly, this deviation can also be interpreted as a sign of LFU violation, since beta decays involve electrons while the most precise determination of Vus comes from decays with final-state muons.
Here, a new-physics effect at a relative sub-per-mille level compared to the SM would suffice to explain the anomaly. This could be achieved by a heavy new lepton or a massive gauge boson affecting the determination of the Fermi constant that parametrises the strength of the weak interactions. As the Fermi constant can also be determined from the global electroweak fit, for which Z decays are crucial inputs, FCC-ee would again be the perfect machine to investigate this anomaly, as it could improve the precision by a large factor (see “High precision” figure). Indeed, the Fermi constant may be determined directly to one part in 105 from the enormous sample (> 1011) of Z decays to tau leptons.
For this dream scenario to play out, at least one beyond-the-SM particle must exist within FCC’s discovery reach
FCC-ee’s extraordinarily large dataset will also enable scrutiny of a long-standing anomaly in the forward-backward asymmetry of Z → bb– decays. The LEP measurement of ΔAFB, which arises from the difference between the Z boson couplings to left- and right-handed chiral states with different strengths, lies 2–3σ below the SM prediction. Although not significant, this anomaly may also be linked to new physics entering in b → s transitions.
Finally, a possible difference in the decay asymmetries of B → D*μν vs B → D*eν was recently reported by an analysis of Belle data. As in the case of RK, the SM prediction that the difference between the muon and the electron asymmetries should be zero is very clean and, like RD and RD*, this observable points towards new physics in b → c transitions and could be related via leptoquarks to g-2 of the muon. Once more, the great number of b quarks to be produced at FCC-ee, together with the clean environment of a lepton collider, would allow this observable to be determined with unprecedented accuracy.
Since all these anomalies point, to varying degrees, towards the existence of LFU-violating new physics, it raises the question of whether a common explanation exists? There are several particularly interesting possibilities, including leptoquarks, new scalars and fermions (as arise in supersymmetric extensions of the SM), new vector bosons (W′ and Z′) and new heavy fermions. In the overwhelming majority of such scenarios, a direct discovery of a new particle is possible at FCC-hh. For example, it could discover leptoquarks with masses up to 10 TeV and Z′ bosons with masses up to 40 TeV, covering most of the mass ranges expected in such models.

A return to the Z pole and beyond
The LEP programme was extremely successful in determining the mechanism of electroweak symmetry breaking, in particular by measuring the properties and decays of the Z boson very precisely from a 17 million-strong sample. This allowed for a prediction of a range for the Higgs mass within which it was later discovered at the LHC. The flavour anomalies could lead to a similar situation in the near future. In this case, the roughly 5 × 1012 Z bosons that the FCC-ee is designed to collect would not only be able to test the effects of new particles in precision electroweak observables, but also, via Z decays into bottom quarks and tau leptons, provide a unique testing ground for flavour physics. As noted earlier, FCC-ee’s Z-pole run is also envisaged to be the first step in a broader electroweak programme encompassing large statistics at the WW and tt– thresholds, in addition to its key role as a precision Higgs factory.
Looking much further ahead to the energy frontier, FCC-hh would be able, in the overwhelming number of scenarios motivated by the flavour anomalies, to directly discover a new particle. Furthermore, FCC-hh would allow for a precise determination of rare Higgs decays and the Higgs potential, probing new-physics effects related to this sector, such as leptoquark explanations of the anomalous magnetic moment of the muon.
Pending the outcome of the FCC feasibility study recommended by the 2020 update of the European strategy for particle physics, the hope that the LEP/LHC success story could be repeated by FCC-ee/FCC-hh is well justified. While FCC-ee could be used to indirectly pin down the parameters of the model(s) of new physics explaining the flavour anomalies via precision electroweak and flavour measurements, FCC-hh would be capable of searching for the predicted particles directly.