A collaboration between CERN and Lausanne University Hospital will see technology developed for the proposed Compact Linear Collider (CLIC) drive a novel cancer radiotherapy facility.
Warning: Undefined array key "attribution" in /opt/app-root/src/wp-content/themes/iopp/inc/shortcodes.php on line 57
Warning: Undefined array key "attribution" in /opt/app-root/src/wp-content/themes/iopp/inc/shortcodes.php on line 57
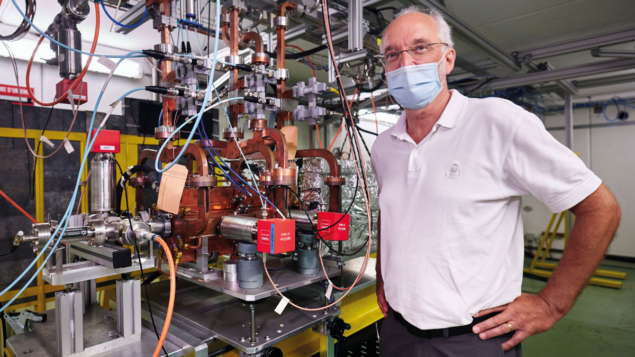
About 30–40% of people will develop cancer during their lifetimes. Surgery, chemotherapy, immunotherapy and radiotherapy (RT) are used to cure or manage the disease. But around a third of cancers are multi-resistant to all forms of therapies, defining a need for more efficient and better tolerated treatments. Technological advances in the past decade or so have transformed RT into a precise and powerful treatment for cancer patients. Nevertheless, the treatment of radiation-resistant tumours is complicated by the need to limit doses to surrounding normal tissue.
A paradigm-shifting technique called FLASH therapy, which is able to deliver doses of radiation in milliseconds instead of minutes as for conventional RT, is opening new avenues for more effective and less toxic RT. Pre-clinical studies have shown that the extremely short exposure time of FLASH therapy spares healthy tissue from the hazardous effect of radiation without reducing its efficacy on tumours.
First studied in the 1970s, it is only during the past few years that FLASH therapy has caught the attention of oncologists. The catalyst was a 2014 study carried out by researchers from Lausanne University Hospital (CHUV), Switzerland, and from the Institute Curie in Paris, which showed an outstanding differential FLASH effect between tumours and normal tissues in mice. The results were later confirmed by several other leading institutes. Then, in 2019, CHUV used FLASH to treat a multi-resistant skin cancer in a human patient, causing the tumour to completely disappear with nearly no side effects.
The consistency of pre-clinical data showing a striking protection of normal tissues with FLASH compared to conventional RT offers a new opportunity to improve cancer treatment, especially for multi-resistant tumours. The very short “radiation beam-on-time” of FLASH therapy could also eliminate the need for motion management, which is currently necessary when irradiating tumours that move with respiration. Furthermore, since FLASH therapy operates best with high single doses, it requires only one or two RT sessions as opposed to multiple sessions over a period of several weeks in the case of conventional RT. This promises to reduce oncology workloads and patient waiting lists, while improving treatment access in low-population density environments. Altogether, these advantages could turn FLASH therapy into a powerful new tool for cancer treatment, providing a better quality of life for patients.
The key requirements for CLIC correspond astonishingly well with the requirements for a FLASH facility
CERN and CHUV join forces
CHUV is undertaking a comprehensive research program to translate FLASH therapy to a clinical environment. No clinical prototype is currently available for treating patients with FLASH therapy, especially for deep-seated tumours. Such treatments require very high-energy beams (see p12) and face technological challenges that can currently be solved only by a very limited number of institutions worldwide. As the world’s largest particle-physics laboratory, CERN is one of them. In 2019, CHUV and CERN joined forces with the aim of building a high-energy, clinical FLASH facility.
The need to deliver a full treatment dose over a large area in a short period of time demands an accelerator that can produce a high-intensity beam. Amongst the current radiation tools available for RT – X-rays, electrons, protons and ions – electrons stand out for their unique combination of attributes. Electrons with an energy of around 100 MeV penetrate many tens of centimetres in tissue so have the potential to reach tumours deep inside the body. This is also true for the other radiation modalities but it is technically simpler to produce intense beams of electrons. For example, electron beams are routinely used to produce X-rays in imaging systems such as CT scanners and in industrial applications such as electron beam-welding machines. In addition, it is comparatively simple to accelerate electrons in linear accelerators and guide them using modest magnets. A FLASH-therapy facility based on 100 MeV-range electrons is therefore a highly compelling option.
Demonstrating the unexpected practical benefits of fundamental research, the emergence of FLASH therapy as a potentially major clinical advance coincides with the maturing of accelerator technology developed for the CLIC electron–positron collider. In a further coincidence, the focus of FLASH development has been at CHUV, in Lausanne, and CLIC development at CERN, in Geneva, just 60 km away. CLIC is one of the potential options for a post-LHC collider and the design of the facility, as well as the development of key technologies, has been underway for more than 20 years. A recent update of the design, now optimized for a 380 GeV initial-energy stage, and updated prototype testing were completed in 2018.
Despite the differences in scale and application, the key requirements for CLIC correspond astonishingly well with the requirements for a FLASH facility. First, CLIC requires high-luminosity collisions, for example to allow the study of rare interaction processes. This is achieved by colliding very high-intensity and precisely controlled beams: the average current during a pulse of CLIC is 1 A and the linac hardware is designed to allow two beams less than 1 nm in diameter to collide at the interaction point. High levels of current that are superbly controlled are also needed for FLASH to cover large tumours in short times. Second, CLIC requires a high accelerating gradient (72 MV/m in the initial stage) to achieve its required collision energy in a reasonably sized facility (11 km for a 380 GeV first stage). A FLASH facility using 100 MeV electrons based on an optimised implementation of the same technology requires an accelerator of just a couple of metres long. Other system elements such as diagnostics, beam shaping and delivery as well as radiation shielding make the footprint of the full facility somewhat larger. Overall, however, the compact accelerator technology developed for CLIC gives the possibility of clinical facilities built within the confines of typical hospital campus and integrated with existing oncology departments.
Over the decades, CLIC has invested significant resources into developing its high-current and high-gradient technology. Numerous high-power radio-frequency test stands have been built and operated, serving as prototypes for the radio-frequency system units that make up a linear accelerator. The high-current-beam test accelerator “CTF3” enabled beam dynamic simulation codes to be benchmarked and the formation, manipulation and control of very intense electron beams to be demonstrated. Further beam-dynamics validations and relevant experiments have been carried out at different laboratories including ATF2 at KEK, FACET at SLAC and ATF at Argonne. CERN also operates the Linear Electron Accelerator for Research (CLEAR) facility, where it can accelerate electrons up to 250 MeV, thus matching the energy requirements of FLASH radiotherapy. For the past several years, and beyond the collaboration between CERN and CHUV, the CLEAR facility has been involved in dosimetry studies for FLASH radiotherapy.
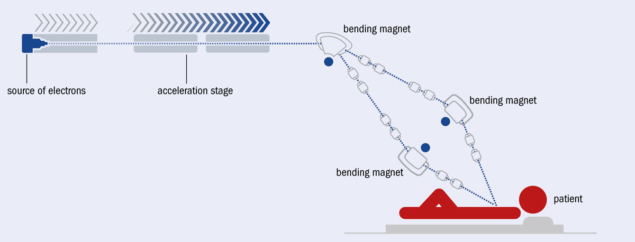
Towards a clinical facility
All of this accumulated experience and expertise is now being used to design and construct a FLASH facility. The collaboration between CERN and CHUV is a shining example of knowledge transfer, where technology developed for fundamental research is used to develop a therapeutic facility. While the technical aspects of the project have been defined via exchanges between medical researchers and accelerator experts, the CERN knowledge-transfer group and CHUV’s management have addressed contractual aspects and identified a strategy for intellectual property ownership. This global approach provides a clear roadmap for transforming the conceptual facility into a clinical reality. From the perspective of high-energy physics, the adoption of CLIC technology in commercially supplied medical facilities would significantly reduce technological risk and increase the industrial supplier base.
An interdisciplinary team comprising medical doctors, medical physicists, radiation biologists and accelerator physicists and engineers was formed
The collaboration between CHUV and CERN was catalysed by a workshop on FLASH therapy hosted by CHUV in September 2018, when it was realised that an electron-beam facility based on CLIC technology offers the possibility for a high-performance clinical FLASH facility. An interdisciplinary team comprising medical doctors, medical physicists, radiation biologists and accelerator physicists and engineers was formed to study the possibilities in greater depth. In an intense exchange during the months following the workshop, where requirements and capabilities were brought together and balanced, a clear picture of the parameters of a clinical FLASH facility emerged. Subsequently, the team studied critical issues in detail, validating that such a facility is in fact feasible. It is now working towards the details of a baseline design, with parameters specified at the system level, and the implementation of entirely new perspectives that were triggered by the study. A conceptual design report for the facility will be finished by the end of 2020. CHUV is actively seeking funding for the facility, which would require approximately three years for construction through beam commissioning.
The basic accelerator elements of the 100 MeV-range FLASH facility that emerged from this design process consist of: a photo-injector electron source; a linac optimised for high-current transport and maximum radio-frequency-power to beam-energy-transfer efficiency; and a beam-delivery system which forms the beam shape for individual treatment and directs it towards the patient. In addition, accelerator and clinical instrumentation are being designed which must work together to provide the necessary level of precision and repeatability required for patient treatment. This latter issue is of particular criticality in FLASH treatment, which must be administered with all feedback and correction of delivered dose to clinical levels completed in substantially less than a second. The radiation field is one area where the requirements of CLIC and FLASH are quite different. In CLIC the beam is focused to a very small spot (roughly 150 nm wide and 3 nm high) for maximum luminosity, whereas in FLASH the beam must be expanded to cover a large area (up to 10 cm) of irregular cross section and with high levels of dose uniformity. Although this requires a very different implementation of the beam-delivery systems, both CLIC and FLASH are designed using the same beam-dynamics tools and design methodologies.
Many challenges will have to be overcome, not least obtaining regulatory approval for such a novel system, but we are convinced that the fundamental ideas are sound and that the goal is within reach. A clinical FLASH facility based on CLIC technology is set to be an excellent example of the impact of developments made in the pursuit of fundamental science can have in society.