LEP was the highest energy e+e– collider ever built, with levels of precision that remain unsurpassed in accelerator physics. Former CERN director of accelerators Steve Myers tells LEP’s story from conception to its emotional final day.
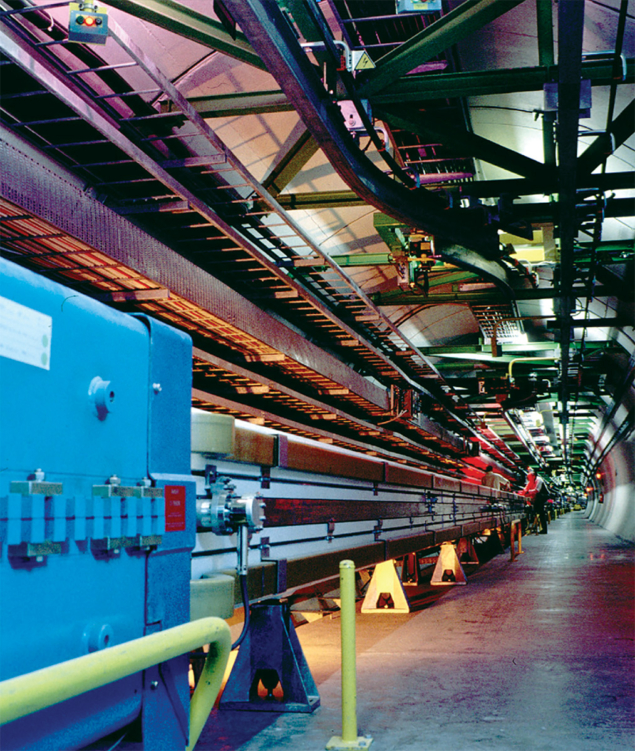
A few minutes before midnight on a summer’s evening in July 1989, 30 or so people were crammed into a back room at CERN’s Prévessin site in the French countryside. After years of painstaking design and construction, we were charged with breathing life into the largest particle accelerator ever built. The ring was complete, the aperture finally clear and the positron beam made a full turn on our first attempt. Minutes later beams were circulating, and a month later the first Z boson event was observed. Here began a remarkable journey that firmly established the still indefatigable Standard Model of particle physics.
So, what can go wrong when you’re operating 27 kilometres of particle accelerator, with ultra-relativistic leptons whizzing around the ring 11,250 times a second? The list is long. The LEP ring was packed with magnets, power converters, a vacuum system, a control system, a cryogenics system, a cooling and ventilation system, beam instrumentation – and much more. Then there was the control system, fibres, networks, routers, gateways, software, databases, separators, kickers, beam dump, radio-frequency (RF) cavities, klystrons, high-voltage systems, interlocks, synchronisation, timing, feedback… And, of course, the experiments, the experimenters and everybody’s ability to get along in a high-pressure environment.
LEP wasn’t the only game in town. There was fierce competition from the more innovative Stanford Linear Collider (SLC) in California. But LEP was off to a fantastic start and its luminosity increase was much faster than at its relatively untested linear counterpart. A short article capturing the transatlantic rivalry appeared in the Economist on 19 August 1989. “The results from California are impressive,” the magazine reported, “especially as they come from a new and unique type of machine. They may provide a sure answer to the generation problem before LEP does. This explains the haste with which the finishing touches have been applied to LEP. The 27 km-long device, six years in the making, was transformed from inert hardware to working machine in just four weeks – a prodigious feat, unthinkable anywhere but at CERN. Even so, it was still not as quick as Carlo Rubbia, CERN’s domineering director-general might have liked.”
Notes from the underground
LEP’s design dates from the late 1970s, the project being led by accelerator-theory group leader Eberhard Keil, RF group leader Wolfgang Schnell and C J “Kees” Zilverschoon. The first decision to be made was the circumference of the tunnel, with four options on the table: a 30 km ring that went deep into the Jura mountains, a 22 km ring that avoided them entirely, and two variants with a length of 26.7 km that grazed the outskirts of the mountains. Then director-general Herwig Schopper decided on a circumference of 26.7 km with an eye on a future proton collider for which it would be “decisive to have as large a tunnel as possible” (CERN Courier July/August 2019 p39). The final design was approved on 30 October 1981 with Emilio Picasso leading the project. Construction of the tunnel started in 1983, after a standard public enquiry in France.
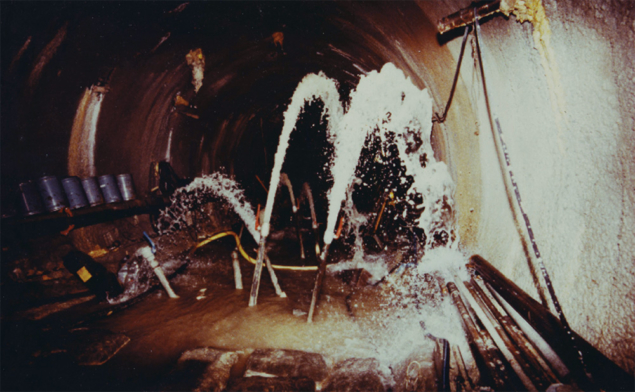
LEP’s tunnel, the longest-ever attempted prior to the Channel Tunnel, which links France and Britain, was carved by three tunnel-boring machines. Disaster struck just two kilometres into the three-kilometre stretch of tunnel in the foothills of the Jura, where the rock had to be blasted because it was not suitable for boring. Water burst in and formed an underground river that took six months to eliminate (figure 1). By June 1987, however, part of the tunnel was complete and ready for the accelerator to be installed.
Just five months after the difficult excavation under the Jura, one eighth of the accelerator (octant 8) had been completely installed, and, a few minutes before midnight on 12 July 1988, four bunches of positrons made the first successful journey from the town of Meyrin in Switzerland (point 1) to the village of Sergy in France (point 2), a distance of 2.5 km. Crucially, the “octant test” revealed a significant betatron coupling between the transverse planes: a thin magnetised nickel layer inside the vacuum chambers was causing interference between the horizontal and vertical focusing of the beams. The quadrupole magnets were adjusted to prevent a resonant reinforcement of the effect each turn, and the nickel was eventually demagnetised.
Giving birth to LEP
The following months saw a huge effort to install equipment in the remaining 24 km of the tunnel – magnets, vacuum chambers and RF cavities, as well as beam instrumentation, injection equipment, electrostatic separators, electrical cabling, water cooling, ventilation and all the rest. This was followed by conditioning the cavities, baking out and leak-testing the vacuum chambers, and individual testing. At the same time a great deal of effort to prepare the software needed to operate the collider was made with limited resources.
In the late 1980s, control systems for accelerators were going through a major transition to the PC. LEP was caught up in the mess and there were many differences of opinion on how to design LEP’s control system. As July 1989 approached, the control system was not ready and a small team was recruited to implement the bare minimum controls required to inject beam and ramp up the energy. Unable to hone key parameters such as the tune and orbit corrections before beam was injected, we had two major concerns: is the beam aperture clear of all obstacles, and are there any polarity errors in the connections of the many thousand magnetic elements? So we nominated a “Mr Polarity”, whose job was to check all polarities in the ring. This may sound trivial, but with thousands of connections it was a huge task.
Tidal forces, melting ice and the TGV to Paris
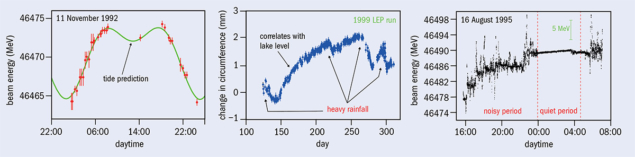
LEP’s beam-energy resolution was so precise that it was possible to observe distortion of the 27 km ring by a single millimetre, whether due to the tidal forces of the Sun and Moon, or the seasonal distortion caused by rain and meltwater from the nearby mountains filling up Lac Léman and weighing down one side of the ring. In 1993 we noticed even more peculiar random variations on the energy signal during the day – with the exception of a few hours in the middle of the night when the signal was noise free. Everybody had their own pet theory. I believed it was some sort of effect coming from planes interacting with the electrical supply cables. Some nights later I could be seen sitting in a car park on the Jura at 2 a.m., trying to prove my theory with visual observations, but it was very dark and all the planes had stopped landing several hours beforehand. Experiment inconclusive! The real culprit, the TGV (a high-speed train), was discovered by accident a few weeks later during a discussion with a railway engineer: leakage currents on the French rail track flowed through the LEP vacuum chamber with the return path via the Versoix river back to Cornavin. The noise hadn’t been evident when we first measured the beam energy as TGV workers had been on strike.
At a quarter to midnight on 14 July 1989, the aperture was free of obstacles and the beam made its first turn on our first attempt. Soon afterwards we managed to achieve a circulating beam, and we were ready to fine tune the multitude of parameters needed to prepare the beams for physics.
The goal for the first phase of LEP was electron–positron collisions at a total energy of 91 GeV – the mass of the neutral carrier of the weak force, the Z boson. LEP was to be a true Z factory, delivering millions of Zs for precision tests of the Standard Model. To mass-produce them required beams not only of high energy but also of high intensity, and delivering them required four steps. The first was to accumulate the highest possible beam current at 20 GeV – the injection energy. This was a major operation in itself, involving LEP’s purpose-built injection linac and electron–positron accumulator, the Proton Synchrotron, the Super Proton Synchrotron (SPS) and, finally, transfer lines to inject electrons and positrons in opposite directions – these curved not only horizontally but also vertically as LEP and the SPS were at different heights. The second step was to ramp up the accumulated current to the energy of the Z resonance with minimal losses. Thirdly, the beam had to be “squeezed” to improve the collision rate at the interaction regions by changing the focusing of the quadrupoles on either side of the experiments, thereby reducing the transverse cross section of the beam at the collision points.
Following the highly successful first turn on 14 July 1989, we spent the next month preparing for the first physics run. Exactly a month later, on 13 August, the beams collided for the first time. The following 10 minutes seemed like an eternity since none of the four experiments – ALEPH, DELPHI, L3 and OPAL – reported any events. I was in the control room with Emilio Picasso and we were beginning to doubt that the beams were actually colliding when Aldo Michelini called from OPAL with the long-awaited comment: “We have the first Z0!” ALEPH and OPAL physicists had connected the Z signal to a bell that sounded on the arrival of the particle in their detectors. While OPAL’s bell rang proudly, ALEPH’s was silent, leading to a barrage of complaints before it became apparent that they were waiting for the collimators to close before turning on their sub detectors. As the luminosity rose during the subsequent period of machine studies the bells became extremely annoying and were switched off.
From the Z pole to the WW threshold

The first physics run began on 20 September 1989, with LEP’s total energy tuned for five days to the Z mass peak at 91 GeV, providing enough integrated luminosity to generate 1400 Zs in each experiment. A second period followed, this time with the energy scanned through the width of the Z at five different beam energies: at the peak and ±1 GeV and ±2 GeV to either side, allowing the experiments to measure the width of the Z resonance. First physics results were announced on 13 October, just three months after the final testing of the accelerator’s components (see LEP’s electroweak leap).
LEP dwelt at the Z peak from 1989 to 1995, during which time the four experiments each observed approximately 4.5 million Z decays. In 1995 a major upgrade dubbed LEP2 saw the installation of 288 superconducting cavities (figure 2), enabling LEP to sit at or near the WW threshold of 161 GeV for the following five years. The maximum beam energy reached was 104.4 GeV. There was also a continuous effort to increase the luminosity by increasing the number of bunches, reducing the emittance by adjusting the focusing, and squeezing the bunches more tightly at the interaction points, with LEP’s performance ultimately limited by the nonlinear forces of the beam–beam interaction – the perturbations of the beams as they cross the opposing beam. LEP surpassed every one of its design parameters (figure 3).
Life as a LEP accelerator physicist
Being an accelerator physicist at LEP took heart as well as brains. The sisyphean daily task of coaxing the seemingly temperamental machine to optimal performance even led us to develop an emotional attachment to it. Challenges were unpredictable, such as for the engineers dispatched on a fact-finding mission to ascertain the cause of an electrical short circuit, only to discover two deer, “Romeo and Juliet”, locked in a lover’s embrace having bitten through a cable, or the discovery of sabotage with beer bottles (see The bizarre episode of the bottles in the beampipe). The aim, however, was clear: inject as much current as possible into both beams, ramp the energy up to 45 GeV, squeeze the beam size down at the collision points, collide and then spend a few hours delivering events to the experiments. The reality was hours of furious concentration, optimisation, and, in the early days, frustrating disappointment.
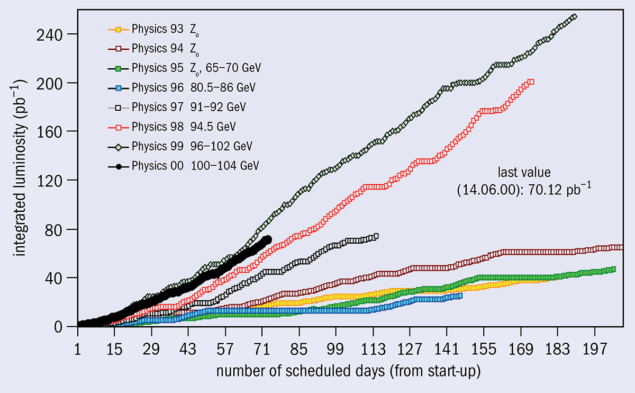
In the early years, filling LEP was a delicate hour-long process of parameter adjustment, tweaking and coaxing the beam into the machine. On a good day we would see the beam wobble alarmingly on the UV telescopes, lose a bit and watch the rest struggle up the ramp. On a bad day, futile attempt after futile attempt, most of the beam would disappear without warning in the first few seconds of the ramp. The process used to last minutes and there was nothing you could do. We would stand there, watching the lifetime buck and dip, and the painstakingly injected beam would either slowly or quickly drift out of the machine. The price of failure was a turn around and refill. Success brought the opportunity to chance the squeeze – an equally hazardous manoeuvre whereby the interaction-point focusing magnets were adjusted to reduce the beam size – and then perhaps a physics fill, and a period of relative calm. At this stage the focus would move to the experimental particle physicists on shift at the four experiments. Each had their own particular collective character, and their own way of dealing with us. We verged between being accommodating, belligerent, maverick, dedicated, professional and very occasionally hopelessly amateur – sometimes all within the span of a single shift, depending on the attendant pressures.

The experiment teams paraded their operational efficiency numbers – plus complaints or congratulations – at twice weekly scheduling meetings. Well run and disciplined, ALEPH almost always had the highest efficiency figures; their appearances at scheduling meetings nearly always a simple statement of 97.8% or thereabouts. This was livened in later years by the repeated appearance of their coordinator Bolek Pietrzyk, who congratulated us each time we stepped up in energy or luminosity with a strong, Polish-accented, “Congratulations! You have achieved the highest energy electron–positron collisions in the universe!”, which was always gratifying. Equally professional, but more relaxed, was OPAL, which had a strong British and German contingent. These guys understood human nature. Quite simply, they bribed us. Every time we passed a luminosity target or hit a new energy record they’d turn up in the control room with champagne or crates of German beer. Naturally we’d do anything for them, happily moving heaven and earth to resolve their problems. L3 and DELPHI had their own quirks. DELPHI, for example, ran their detector as a “state machine”, whose status changed automatically based on signals from the accelerator control room. All well and good, but they depended on us to change the mode to “dump beam” at the end of a fill, something that was occasionally skipped, leaving DELPHI’s subdetectors on and them ringing us desperately for a mode change. Baffled DELPHI students on shift would ask what was going on. Filling and ramping were demanding periods during the operational sequence and a lot of concentration was required. The experiment teams did well not to ring and make too many demands at this stage – requests were occasionally rebuffed with a brusque response.
On the verge of a great discovery?
LEP’s days were never fated to dwindle. Early on, CERN had a plan to install the LHC in the same tunnel, in a bid to scan ever higher energies and be the first to discover the Higgs boson. However, on 14 June 2000, LEP’s final year of scheduled running, the ALEPH experiment reported a possible Higgs event during operations at a centre-of-mass energy of 206.7 GeV. It was consistent with “Higgs-strahlung”, whereby a Z radiates a Higgs boson, which was expected to dominate Higgs-boson production in e+e– collisions at LEP2 energies. On 31 July and 21 August ALEPH reported second and third events corresponding to a putative reconstructed Higgs mass in the range 114–115 GeV.
The bizarre episode of the bottles in the beampipe
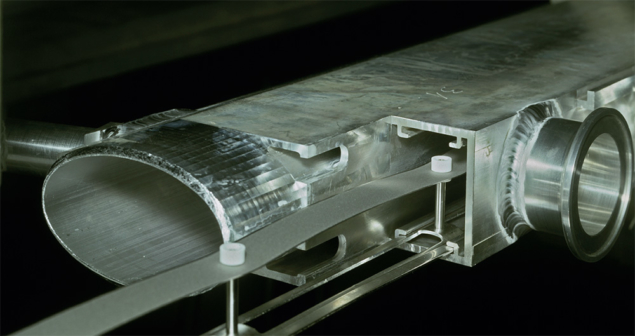
The story of the sabotage of LEP has grown in the retelling, but I was there in June 1996, hurrying back early from a conference to help the machine operators, who had been struggling to circulate a beam for several days. After exhausting other possibilities, it became clear that there was an obstruction in the vacuum pipe, and we detected the location using the beam position system. It appeared to be around point 1 (where ATLAS now sits), so we opened the vacuum seal and took a look inside the beampipe using mirrors and endoscopes. Not seeing anything, I frustratedly squeezed my head between the vacuum flanges and peered down inside the pipe. In the distance was something resembling a green concave lens. “This looks like the bottom of a beer bottle,” I thought, restraining myself from uttering a word to anyone in the vicinity. I went to the opposite open end of the vacuum section and peered into the vacuum pipe again: a green circular disk this time, but again, not a word. Someone got a long pole to poke out the offending article – out it came, and my guess was correct: it was a Heineken beer bottle, which had indeed refreshed the parts no other beer could reach, as the slogan ran. A hasty search revealed a second bottle. Upon closer inspection it was clear that the control room operators had almost succeeded in making the beam circulate despite the obstacles: there was a scorch burn along the label, indicating that they had almost managed to steer the beam past the bottles. If there had only been one they may have succeeded. The Swiss police interviewed me concerning this act of sabotage but the culprit was never unmasked.
LEP was scheduled to stop in mid-September with two weeks of reserve time granted to the LEP experiments to see if new Higgs-like events would appear. After the reserve weeks, ALEPH requested two months more running to double its integrated luminosity. One was granted, yielding a 50% increase in the accumulated data, and ALEPH presented an update of their results on 10 October: the signal excess had increased to 2.6σ. Things were really heating up, and on 16 October L3 announced a missing-energy candidate. By now the accelerator team was pushing LEP to its limits, to squeeze out every ounce of physics data in the service of the experiments’ search for the elusive Higgs. At the LEP committee meeting on 3 November, ALEPH presented new data that confirmed their excess once again – it had now grown to 2.9σ. A request to extend LEP running by one year was made to the LEPC. There was gridlock, and no unanimous recommendation could be made.
All of CERN was discussing the proposed running of LEP in 2001 to get final evidence of a possible discovery of the Higgs boson. Arguments against included delays to the start of the LHC of up to three years. There was also concern that Fermilab’s Tevatron would beat the LHC to the discovery of the Higgs, and mundane but practical arguments about the transfer of human resources to the LHC and the impact on the materials budget, including electricity costs. The impending closure of LEP, when many of us thought we were about to discover the Higgs, was perceived like the death of a dear friend by most of the LEP-ers. After each of the public debates on the subject a group of us would meet in some local pub, drink a few beers, curse the disbelievers and cry on each other’s shoulders. This was the only “civil war” that I saw in my 43 years at CERN.
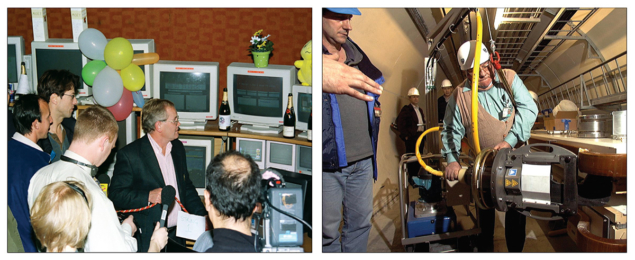
The CERN research board met again on 7 November and again there was deadlock, with the vote split eight votes to eight. The next day, then director-general Luciano Maiani announced that LEP had closed for the last time. It was a deeply unpopular decision, but history has shown it to be correct: the Higgs was discovered at the LHC 12 years later, with a mass of not 115 but 125 GeV. LEP’s closure allowed a massive redeployment of skilled staff, and the experience gained for the first time in running large accelerators went on to prove essential to the safe and efficient operation of the LHC.
When LEP was finally laid to rest we met one last time for an official wake (figure 4). After the machine was dismantled, requiring the removal to the surface of around 30,000 tonnes of material, some of the magnets and RF units were shipped to other labs for use in new projects. Today, LEP’s concrete magnet casings can still be seen scattered around CERN as shielding units for antimatter and fixed-target experiments, and even as road barriers.
LEP was the highest energy e+e– collider ever built. Its legacy was and is extremely important for present and future colliders. The quality and precision of the physics data remain unsurpassed in luminosity, energy and energy calibration. It is the reference for any future e+e–-ring collider design.