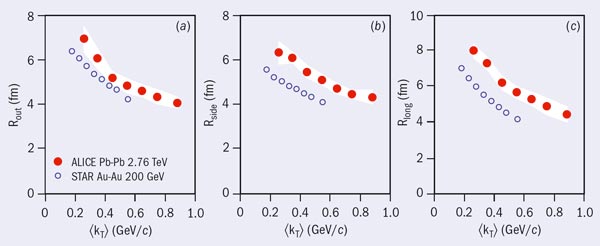
The ALICE collaboration has measured the size of the pion-emitting system in central lead–ion collisions at the LHC at a centre-of-mass energy of 2.76 TeV per nucleon pair. The radii of the pion source were deduced from the shape of the Bose-Einstein peak in the two-pion correlation functions.
In hadron and ion collisions, Bose-Einstein quantum statistics leads to enhanced production of bosons that are close together in phase space, and thus to an excess of pairs at low relative momentum. The width of the excess region is inversely proportional to the system size at decoupling, i.e. at the point when the majority of the particles stop interacting.
An important finding at the Relativistic Heavy Ion Collider (RHIC) at Brookhaven was that the QCD matter created there behaved like a fluid, with strong collective motions that are well described by hydrodynamic equations. The collective flow makes the size of the system appear smaller with increasing momentum of the pair. This behaviour is also clearly visible for the radii measured at the LHC in the ALICE experiment. Figure 1 shows the results for measurements of the radius of the pion source in three dimensions: along the beam axis, Rlong; along the transverse momentum (kT) of the pair, Rout; and in a direction perpendicular to these two, Rside.
The similarity between the values for Rout and Rside indicates a short duration for the emission, hence an “explosive” emission. The time when the emission reaches its maximum – measured with respect to the first encounter – can be derived from the dependence of the longitudinal radius on the transverse momentum, Rlong(kT). ALICE has found this to be 10–11 fm/c, which is significantly longer than it is at RHIC. Moreover, the product of the three radii at low pair-momentum – the best estimate of the homogeneity volume of the system at decoupling – is twice as large as at RHIC (figure 2).

These results, taken together with those obtained from the study of the multiplicity and the azimuthal anisotropy, indicate that the fireball formed in nuclear collisions at the LHC is hotter, lives longer and expands to a larger size than at lower energies. Further analyses, in particular including the full dependence of these observables on centrality, will provide more insights into the properties of the system – such as initial velocities, the equation of state and the fluid viscosity – and strongly constrain the theoretical modelling of heavy-ion collisions.