In analysing data from last year’s test run with proton–lead collisions in the LHC, the ALICE collaboration, followed almost immediately and independently by the ATLAS collaboration, have announced a surprising observation in the way that particles emerge from the high-energy collisions. Here the two collaborations report on their results.

To prepare for the recent LHC run with collisions of protons and lead ions, the LHC team performed a test run for a few hours last September. During this run the ALICE experiment recorded close to two million events, which have already led to new results (CERN Courier December 2012 p6). Now, after an in-depth analysis, the ALICE collaboration has made the surprising observation of a double “ridge” structure in the correlation of particles emerging from the proton–lead collisions. This follows the observation by the CMS collaboration, using data from the same test run, of a “near-side” ridge-like correlation structure elongated in pseudorapidity – a measure of the angle an emerging particle takes relative to the direction of the beam (CERN Courier January/February 2013 p9).
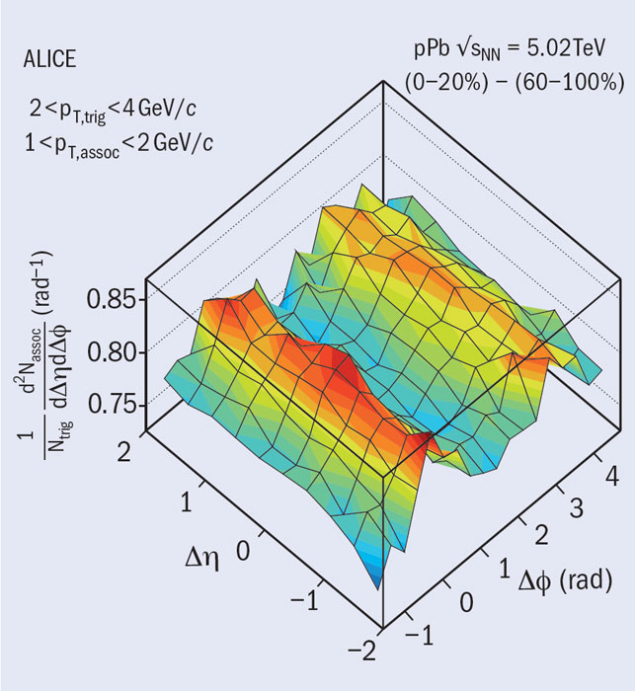
The analysis performed by the ALICE collaboration characterizes two-particle angular correlations as a function of the event activity, which is quantified by the multiplicity, measured in a pair of forward scintillator detectors. The correlations are determined by counting the number of associated particles as a function of their difference in azimuth (Δφ) and pseudorapidity (Δη) with respect to a trigger particle, in bins of this particle’s transverse momentum pT, trig and associated transverse momentum pT,assoc.
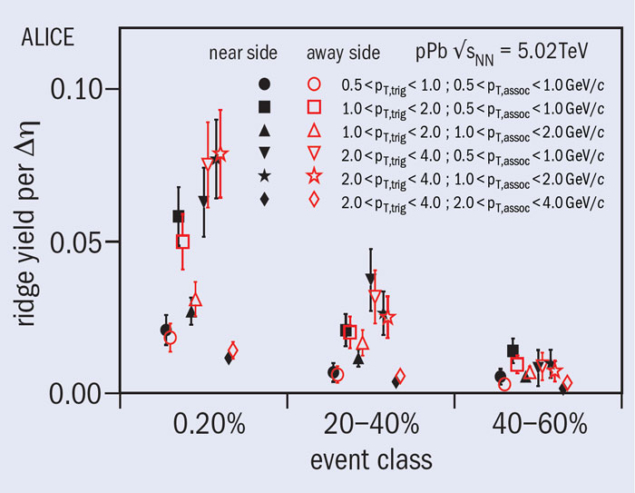
On the “near side” (Δφ≈0), the separation of an Δη-elongated ridge structure from the contribution of a jet to the correlation is straightforward because the jet peak is concentrated around Δη= 0. It is more difficult on the “away side” (Δφ≈π) because both structures are elongated in Δη and not easily separable by selecting on Δη. Experimentally, however, the near-side jet peak shows only a weak evolution with event multiplicity. So by subtracting the correlations at different event multiplicities from one another, it is possible to remove the jet-like contribution of the correlation to a large extent and to quantify modifications as a function of event multiplicity.
Figure 1 shows the two-particle correlation of low-multiplicity events subtracted from that of high-multiplicity events. It reveals a distinct excess in the correlation, which forms two ridges along Δη. The ridge on the near side, qualitatively similar to the one observed by CMS, is accompanied by a second ridge of similar magnitude on the away side, which is observed for the first time.
Such double-ridge structures are typically found in collisions of heavy ions and have their origins in collective phenomena occurring in the quark–gluon plasma that is created. However, these phenomena are not generally thought to occur in proton–lead collisions, where the size of the collision region is expected to be too small to allow the development of significant collective effects.
The projection of figure 1 onto Δφ allows the yield and width of the near-side and away-side ridges to be quantified above a constant baseline. Figure 2 presents the ridge yield for different event multiplicities. It is remarkable that the near- and away-side yields always agree within uncertainties for a given sample despite the absolute values changing substantially with event multiplicity and pT interval. Such a tight correlation between the yields suggests a common underlying physical origin for the two ridges. The extracted widths on the near side and the away side agree with each other within 20% and show no significant dependence on pT, which suggests that the observed ridge is not of jet origin.
This intriguing and unexpected result still needs to be explained theoretically. Models that produce almost identical near- and away-side ridges are based on the colour-glass condensate framework or on hydrodynamical calculations that assume collective effects to occur also in proton–lead collisions. Whatever the origin may be, this observation has opened the window on a novel phenomenon. Further analysis of the high-statistics proton–lead data promises to yield exciting results.
Further reading
ALICE collaboration 2013 Phys. Lett. B 719 29.

Studies of two-particle correlations in high-multiplicity proton–proton and proton–lead collisions at the LHC have shown a phenomenon frequently referred to as the “ridge”. The ridge is a result of correlated production of particles at small relative-azimuthal angle (Δφ) over a wide range of relative pseudorapidity (Δη). Using data from the highly successful pilot proton–lead run on 12 September 2012, ATLAS has shown that the ridge has an identical twin resulting from correlated production of particles that are back-to-back in azimuth.
To observe this twin, ATLAS had to remove background in the two-particle correlation function arising from hard scattering processes, momentum conservation and low-momentum resonance decays. Two-particle correlations were measured as a function of the proton–lead total transverse energy (ΣET) detected in one of the ATLAS forward calorimeters. The contribution of the background to the two-particle correlations was found to be independent of ΣET. As a result, the background could be measured in low-ΣET proton–lead collisions, which have little contribution from the ridge, and then subtracted from the two-particle correlation function in high-ΣET collisions.

The left and right panels in the figure (above) show the two-particle correlation function before and after background subtraction, respectively. Before subtraction, the correlation function includes: a jet peak near Δφ = 0, Δη = 0; the previously observed ridge; and a broad structure arising from particles recoiling from the jet. The subtraction procedure removes the recoil contribution and nearly all of the jet peak, leaving behind two symmetrical ridges, extending over +/–5 units of Δη. The strength of the correlation increases with the transverse momentum of the particles over the measured pT range, 0 < pT < 6 GeV.
The presence of such a symmetrical ridge had been predicted by QCD calculations invoking the colour-glass condensate, which describes the gluon content of a high-energy nucleus in the saturation regime. Alternative calculations that model the system formed in proton–lead collision as a “near perfect fluid” have also predicted a symmetrical ridge arising from final-state collective motion similar to that observed in lead–lead collisions. The data collected during the recent 2013 high-luminosity proton–lead run should provide a way to resolve this theoretical ambiguity. The good news is that either explanation will represent a ground-breaking advance in the understanding of high-energy proton–nucleus collisions.
Further reading
ATLAS collaboration 2012 arXiv:1212.5198 [hep-ex], submitted to Phys. Rev. Lett.