Forty years after its discovery, the W boson continues to intrigue. Chris Hays describes recent progress in understanding a surprisingly high measurement of its mass using data from the former CDF experiment.
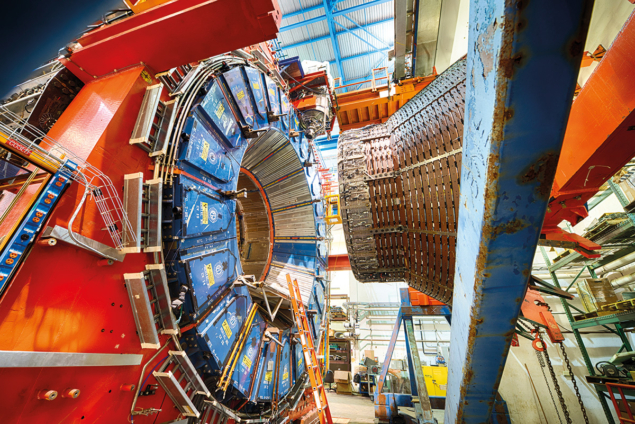
The discovery of the W boson at CERN in 1983 can well be considered the birth of precision electroweak physics. Measurements of the W boson’s couplings and mass have become ever more precise, progressively weaving in knowledge of other particle properties through quantum corrections. Just over a decade ago, the combination of several Standard Model (SM) parameters with measurements of the W-boson mass led to a prediction of a relatively low Higgs-boson mass, of order 100 GeV, prior to its discovery. The discovery of the Higgs boson in 2012 with a mass of about 125 GeV was hailed as a triumph of the SM. Last year, however, an unexpectedly high value of the W-boson mass measured by the CDF experiment threw a spanner into the works. One might say the 40-year-old W boson encountered a midlife crisis.
The mass of the W boson, mW, is important because the SM predicts its value to high precision, in contrast with the masses of the fermions or the Higgs boson. The mass of each fermion is determined by the strength of its interaction with the Brout–Englert–Higgs field, but this strength is currently only known to an accuracy of approximately 10% at best; future measurements from the High-Luminosity LHC and a future e+e– collider are required to achieve percent-level accuracy. Meanwhile, mW is predicted with an accuracy better than 0.01%. At tree level, this mass depends only on the mass of the Z boson and the weak and electromagnetic couplings. The first measurements of mW by the UA1 and UA2 experiments at the SppS collider at CERN were in remarkable agreement with this prediction, within the large uncertainties. Further measurements at the Tevatron at Fermilab and the Large Electron Positron collider (LEP) at CERN achieved sufficient precision to probe the presence of higher-order electroweak corrections, such as from a loop containing top and bottom quarks.
Increasing sophistication
Measurements of mW at the four LEP experiments were performed in collisions producing two W bosons. Hadron colliders, by contrast, can produce a single W-boson resonance, simplifying the measurement when utilising the decay to an electron or muon and an associated neutrino. However, this simplification is countered by the complication of the breakup of the hadrons, along with multiple simultaneous hadron–hadron interactions. Measurements at the Tevatron and LHC have required increasing sophistication to model the production and decay of the W boson, as well as the final-state lepton’s interactions in the detectors. The average time between the available datasets and the resulting published measurement have increased from two years for the first CDF measurement in 1991 to more than 10 years for the most recent CDF measurement announced last year (CERN Courier May/June 2022 p9). The latter benefitted from a factor of four more W bosons than the previous measurement, but suffered from a higher number of additional simultaneous interactions. The challenge of modelling these interactions while also increasing the measurement precision required many years of detailed study. The end result, mW = 80433.5 ± 9.4 MeV, differs from the SM prediction of mW = 80357 ± 6 MeV by approximately seven standard deviations (see “Out of order” figure).

The SM calculation of mW includes corrections from single loops involving fermions or the Higgs boson, as well as from two-loop processes that also include gluons. The splitting of the W boson into a top- and bottom-quark loop produces the largest correction to the mass: for every 1 GeV increase in top-quark mass the predicted W mass increases by a little over 6 MeV. Measurements of the top-quark mass at the Tevatron and LHC have reached a precision of a few hundred MeV, thus contributing an uncertainty on mW of only a couple of MeV. The calculated mW depends only logarithmically on the Higgs-boson mass mH, and given the accuracy of the LHC mH measurements, it contributes negligibly to the uncertainty on mW. The tree-level dependence of mW on the Z-boson mass and on the electromagnetic coupling strength contribute an additional couple of MeV each to the uncertainty. The robust prediction of the SM allows an incisive test through mW measurements, and it would appear to fail in the face of the recent CDF measurement.
Since the release of the CDF result last year, physicists have held extensive and detailed discussions, with a recurring focus on the measurement’s compatibility with the SM prediction and with the measurements of other experiments. Further discussions and workshops have reviewed the suite of Tevatron and LHC measurements, hypothesising effects that could have led to a bias in one or more of the results. These potential effects are subtle, as fundamentally the W-boson signature is strikingly unique and simple: a single charged electron or muon with no observable particle balancing its momentum. Any source of bias would have to lie in a higher-order theoretical or experimental effect, and the analysts have studied and quantified these in great detail.
Progress
In the spring of this year ATLAS contributed an update to the story. The collaboration re-analysed its data from 2011 to apply a comprehensive statistical fit using a profile likelihood, as well as the latest global knowledge of parton distribution functions (PDFs) – which describe the momentum distribution functions of quarks and gluons inside the proton. The preliminary result (mW = 80360 ± 16 MeV) reduces the uncertainty and the central value of its previous result published in 2017, further increasing the tension between the ATLAS result and that of CDF.
Meanwhile, the Tevatron+LHC W-mass combination working group has carried out a detailed investigation of higher-order theoretical effects affecting hadron-collider measurements, and provided a combined mass value using the latest published measurement from each experiment and from LEP. These studies, due to be presented at the European Physical Society High-Energy Physics conference in Hamburg in late August, give a comprehensive and quantitative overview of W-boson mass measurements and their compatibilities. While no significant issues have been identified in the measurement procedures and results, the studies shed significant light on their details and differences.
LHC versus Tevatron
Two important aspects of the Tevatron and LHC measurements are the modelling of the momentum distribution of each parton in the colliding hadrons, and the angular distribution of the W boson’s decay products. The higher energy of the LHC increases the importance of the momentum distributions of gluons and of quarks from the second generation, though these can be constrained using the large samples of W and Z bosons. In addition, the combination of results from centrally produced W bosons at ATLAS with more forward W-boson production at LHCb reduces uncertainties from the PDFs. At the Tevatron, proton–antiproton collisions produced a large majority of W bosons via the valence up and down (anti)quarks inside the (anti)proton, and these are also constrained by measurements at the Tevatron. For the W-boson decay, the calculation is common to the LHC and the Tevatron, and precise measurements of the decay distributions by ATLAS are able to distinguish several calculations used in the experiments.
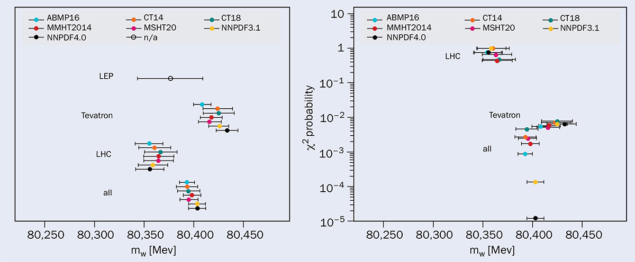
In any combination of measurements, the primary focus is on the uncertainty correlations. In the case of mW, many uncertainties are constrained in situ and are therefore uncorrelated. The most significant source of correlated uncertainty is the PDFs. In order to evaluate these correlations, the combination working group generated large samples of events and produced simplified models of the CDF, DØ and ATLAS detectors. Several sets of PDFs were studied to determine their compatibility with broader W- and Z-boson measurements at hadron colliders. For each of these sets the correlations and combined mW values were determined, opening a panorama view of the impact of PDFs on the measurement (see “Measuring up” figure).
The mass of the W boson is important because the SM predicts its value to high precision, in contrast with the masses of the fermions or the Higgs boson
The first conclusion from this study is that the compatibility of all PDF sets with W- and Z-boson measurements is generally low: the most compatible PDF set, CT18 from the CTEQ collaboration, gives a probability of only 1.5% that the suite of measurements are consistent with the predictions. Using this PDF set for the W-boson mass combination gives an even lower compatibility of 0.5%. When the CDF result is removed, the compatibility of the combined mW value is good (91%), and when comparing this “N-1” combined value to the CDF value for the CT18 set, the difference is 3.6σ. The results are considered unlikely to be compatible, though the possibility cannot be excluded in the absence of an identified bias. If the CDF measurement is removed, the combination yields a mass of mW = 80369.2 ± 13.3 MeV for the CT18 set, while including all measurements results in a mass of mW = 80394.6 ± 11.5 MeV. The former value is consistent with the SM prediction, while the latter value is 2.6σ higher.
Two scenarios
The results of the preliminary combination clearly separate two possible scenarios. In the first, the mW measurements are unbiased and differ due to large fluctuations and the PDF dependence of the W- and Z-boson data. In the second, a bias in one or more of the measurements produces the low compatibility of the measured values. Future measurements will clarify the likelihood of the first scenario, while further studies could identify effect(s) that point to the second scenario. In either case the next milestone will take time due to the exquisite precision that has now been reached, and to the challenges in maintaining analysis teams for the long timescales required to produce a measurement. The W boson’s midlife crisis continues, but with time and effort the golden years will come. We can all look forward to that.
Further reading
LHC-TeV MWWG 2023 arXiv:2308.09417 (submitted to EPJC).
twiki.cern.ch/twiki/bin/view/LHCPhysics/LHC-TEV-MWWG.