
The Sudbury Neutrino Observatory, which started taking data in 1999, has announced its first results on solar neutrinos, which confirm the suspicion that something happens to these particles on their 150 million kilometre journey from the Sun to the Earth.
Experiments have been monitoring solar neutrinos for some 40 years. To see neutrinos at all demands a major effort, so measurements are difficult and reliable results take time to amass. As the work continued, physicists began to suspect that their experiments were not seeing as many solar neutrinos as expected – there was a “solar neutrino problem”.
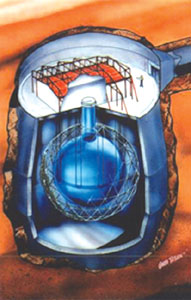
Neutrinos are produced in the nuclear reactions in the Sun’s core, which provide the Sun’s energy (the radiant light and heat which make life possible is only a by-product of the Sun’s nuclear furnace). If physicists think that they understand what happens inside the Sun, they should be able to predict the number of neutrinos which arrive at the Earth. When measurements do not agree with the prediction, there is a dilemma – either we do not understand how the Sun works, or neutrinos are perverse particles that do not behave as expected.
In appraising these two alternatives, it is important to remember that, 100 years ago, physicists could not understand where the Sun got its energy from and why it hadn’t yet burned out. Only the advent of nuclear physics in the 1930s showed how nuclear transformations could supply such prodigious and enduring outputs. The neutrino concept was an initially hesitant postscript to this nuclear picture. To understand nuclear beta decay, there had to be a particle that would be very difficult to detect – if it could be detected at all. From the start, neutrinos acquired a reputation for being non conformist.
The new Sudbury results confirm that bizarre neutrino behaviour is the reason for the solar neutrino deficit – the particles are indeed living up to their non conformist reputation.
Neutrinos come in three types – electron, muon and tau – according to their subnuclear parentage. When such distinct neutrino types were first discovered, it was initially believed that each type was immutable – a neutrino born with an electron (as in beta decay or the reactions deep inside the Sun) could continue to show such electron character for ever.
However, the non conformist reputation of these particles led some far-sighted physicists to suspect that perhaps neutrinos were not immutable. Perhaps there was a small chance that a neutrino could change its allegiance in flight. A neutrino that began its journey in electron class could ‘oscillate’ and upgrade to muon class. Such changed seating arrangements en route could explain an observed deficit of electron-type solar neutrinos.
The Sudbury Neutrino Observatory (SNO) is a vessel containing 1000 tonnes of heavy water, 2000 m underground in an active nickel mine in Ontario, Canada. Particles resulting from neutrino collisions produce flashes of light that are picked up by 9500 photomultiplier tubes. The detector is sensitive to those solar neutrinos produced via the beta decay of boron-8.
The heavy water is the key – SNO is the first extraterrestrial neutrino detector to use heavy water. In one heavy water reaction (call it reaction A), an electron-type neutrino can break up a target deuteron, producing two protons and an emergent electron. Electrons can also appear from elastic scattering (reaction B), where an incoming neutrino bounces off an atomic electron, which then recoils. However, reaction B can be produced by any kind of neutrino.
Over 241 days, SNO collected 1169 neutrino events, which were carefully analysed to classify them as being due to reaction A or B.
The apparent flux of solar neutrinos measured via the observed rate for reaction A (1.75 0. ± 07 + 0.12 – 0.11 ± 0.05 x 106 cm-2 s-1, where the three sets of errors are respectively statistical, systematic and theoretical) is slightly lower than the precision measurement (2.32 ± 0.03 + 0.08 – 0.07 x 106 cm-2 s-1,) via reaction B, by the Superkamiokande detector in Japan (CERN Courier September 2000 p8 – SNO’s measurement of the rate for reaction B has not yet attained this precision). The fluxes as measured via the two reactions are different because some of the electron neutrinos produced in the Sun have “oscillated” into other types of neutrino en route, and on arrival at SNO are no longer able to trigger reaction A.
Evidence for neutrino oscillations has been seen in other situations. The SNO result is the first direct evidence for solar neutrinos oscillating on their journey to Earth. When an experiment makes its debut with such important results, its future looks assured.