An exceptional run for the heavy-ion collider in 2014.

Image credit: Brookhaven National Laboratory.
The Relativistic Heavy Ion Collider (RHIC) at Brookhaven National Laboratory completed its 14th physics run in July, during which gold-ion beams were brought into collision at both low (7.3 GeV/nucleon) and high (100 GeV/nucleon) energies. The runs at high energy set new records for instantaneous and average-store luminosities, and the latter now stands at 5 × 1027 cm–2 s–1, or 25 times the design value. This stellar performance also allowed the introduction of another combination of species to the study of quark–gluon plasma (QGP). For the first time, a collider brought ions of helium-3 – a rare helium isotope with two protons and a single neutron – into collision with gold nuclei.
For the first three weeks of the 2014 run, RHIC delivered gold–gold collisions at 7.3 GeV/nucleon to complete the first phase of a beam-energy scan. The aim of the energy scan is to find a critical point in the QCD phase diagram that marks the end point of a first-order phase transition from cold nuclear matter into QGP. The majority of the scan was done in 2010, with five different collision energies. To date, gold ions have collided with 3.85, 4.6, 5.75, 9.8, 19.5, 27.9, 31.2, 65.2 and 100 GeV/nucleon. A second phase of the beam-energy scan is now planned for energies below the nominal injection energy of 9.8 GeV/nucleon, with a luminosity increase ranging from a factor of three to 10. The large increase in luminosity requires the implementation of electron cooling. Meanwhile, the low-energy part has already allowed the STAR and PHENIX collaborations to test new components in preparation for the high-energy part of the run: the heavy-flavour tracker for STAR and the vertex detector for PHENIX.
The subsequent 18 weeks of the 2014 run with collisions at a beam energy of 100 GeV/nucleon had the goal of delivering as much luminosity as possible, following a luminosity upgrade. This year marked the end of this upgrade period, which began in 2007 and saw the average store luminosity for gold–gold collisions increase by more than a factor of four. The two main elements of the upgrade have been an increase in the bunch intensity from 1.1 × 109 to 1.6 × 109 gold ions, and the implementation of three-dimensional stochastic cooling in both of RHIC’s rings (Blaskiewicz et al. 2008). The increase in the bunch intensity was achieved through many small upgrades in all of the machines, from the source to RHIC (figure 1), and led to a 2.5-fold increase in the initial luminosity. In addition, stochastic cooling led to a luminosity lifetime that is now determined by “burn-off”, with more than 90% of the gold ions lost in collisions with the other beam. Without stochastic cooling, the increased initial luminosity would decay so fast as to be of no use.
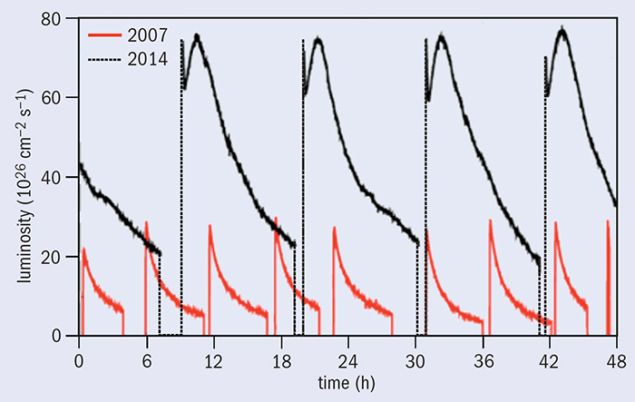
Figure 2 shows the dramatic effect that the upgrades had on the instantaneous and average-store luminosities, where an operating period of 48 h is shown in 2007 and 2014. The 2014 luminosity starts at a much higher value, but still decays for about half an hour before the cooling takes full effect. The cooling then reduces the beam sizes fast enough that the luminosity begins to increase, and typically exceeds the initial value. It then decays with time as more and more ions are lost in the collision process. The 2014 stores ended with luminosity values that are as high as the initial values in 2007.
With the high-luminosity stores and excellent reliability, the integrated luminosity of the 2014 run exceeds the integrated luminosity of all previous gold–gold runs combined. Figure 3 shows the integrated nucleon-pair luminosity, LNN = A1A2L, where L is the integrated luminosity, and A1 and A2 are the number of nucleons of the ions in the two beams, respectively. The use of LNN allows different ion combinations to be compared.
The shutdown period preceding the 2014 run provided the opportunity to upgrade a number of subsystems. Bunch-merging in the Booster and Alternating Gradient Synchrotron (AGS) was improved with a new low-level RF system, leading to an overall increase of about 30% in the maximum extracted beam intensity, compared with the 2012 run, the last time that gold ions were used in RHIC. The RHIC stochastic-cooling system, made fully operational in all three planes for the first time for the 2012 uranium run, featured new longitudinal pick-ups and kickers, for better correction of the spread of particles within the bunches (CERN Courier October 2012 p17). Additionally, a new 56 MHz passive superconducting RF storage cavity was commissioned, to provide larger RF buckets and reduce longitudinal diffusion caused by intra-beam scattering. The cavity is the first superconducting cavity in RHIC, and it reached 300 kV, which is below the 2 MV design voltage because it was limited by quenches in a higher-order mode damper. With a redesign of the damper and the full voltage of the cavity, the average store luminosity is expected to increase even further in the future, by at least 30%.

To minimize the commissioning time of the collider for the 100 GeV gold–gold run, the decision was taken to use the lattice design for the 2012 uranium run. This provides an increased off-momentum dynamic aperture, compared with previous high-energy heavy-ion runs, of up to 5σ for δp/p = 1.8 × 10–4. The machine performance during the 2012 run with uranium–uranium and copper–gold was such that beam losses were already dominated by burn-off (Luo et al. 2014).
Further highlights
One of the highlights of the 2014 run was the implementation of a dynamic β*-squeeze scheme to increase the integrated luminosity delivered to the STAR and PHENIX experiments. The beam size at any given point in the storage ring is given by √βε, where β is the β function and ε is the emittance. In this scheme, the β function at the interaction point is reduced while the beams are in collision. The scheme takes advantage of the fact that, owing to stochastic cooling, the emittance ε decreases during the store, so that a larger β function can be accommodated in the final focus triplets. With this, the β function at the interaction point (β*) – and therefore the beam size – is reduced, leading to an increase in luminosity. After about one hour, the transverse emittance ε is reduced by a factor 2.5–3, and eventually to less than 1 μm rms.
The lattice design for this dynamic squeeze relies on the principles of the achromatic telescopic squeeze (ATS) developed at CERN in the context of the LHC upgrade (Fartoukh 2013). The ATS method was adapted for RHIC to match the machine constraints, both in engineering (the magnet power-supplies’ wiring scheme) and in beam dynamics (the location of experimental insertions and phase-advance requirements). Once the linear optics had been corrected – reducing the β beat in the machine from 40% to 10% – it was possible to ramp the lattice dynamically into its new set point, sending the β* from 0.70 m down to 0.50 m. This could only be done reliably with the help of orbit and tune feedbacks. Prior to their operational implementation, each new β* set point was commissioned during dedicated beam-experiment periods.
Figure 4 shows the luminosity at the STAR detector before, during and after a β squeeze, 7 h into a physics store. The dynamic β squeeze became part of the routine operation in the second half of the 2014 run, and will be the main method to level the luminosity in future. To maximize the physics output, RHIC’s detectors might require that the instantaneous luminosity does not exceed a certain value. With cooling and the dynamic β squeeze, ions can be stored and burned off in the collisions in a way that is most useful to the experiments.
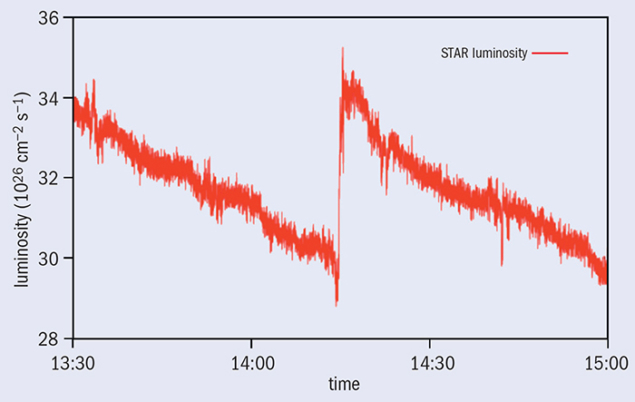
With the success of the gold–gold run at 100 GeV/nucleon, the last weeks of the 2014 run were reassigned to allow a new type of collision to be studied: helium-3 on gold ions. Understanding the properties of QGP can be advanced by looking into the emission patterns of the subatomic particles that it generates while cooling down. These patterns are a function of the initial “shape” of the QGP, which is given by the type of collision between the two stored beams. In colliders such as RHIC and the LHC, almost spherical particles (e.g. protons, gold and lead) are sent to collide head on, resulting in showers of subatomic particles that are projected in a circular pattern. The idea behind using helium-3 is that it features a triangular shape, allowing the STAR and PHENIX collaborations to look at different initial forms of QGP.
The biggest challenge for RHIC was to send the helium-3 beam onto the gold-ion beam for head-on collisions. Given the large difference in the charge-to-mass ratio of the two species – 2:3 for helium-3 versus 79:197 for gold – the beam trajectories were such that it became necessary to add crossing angles through the collision points in STAR and PHENIX, and a large horizontal orbit excursion in both interaction regions. The orbit excursions reached 10 mm – for comparison, well-controlled orbits have rms values of 20 μm. In addition, with a circumference of 3833 m, the path length of the helium-3 beam was 10 mm longer than that of the gold-ion beam.
Thanks to a modified bunch-merging mechanism through the RHIC injector chain, the bunch intensity for the helium-3 beam was increased by a factor of four compared with the previous year. With this significant improvement, the successful implementation of the specific beam paths in all of the interaction regions, and a short commissioning time despite the complexity of running with two particle species that are so different, this dedicated run was also a major success, exceeding the luminosity goals for both experiments.