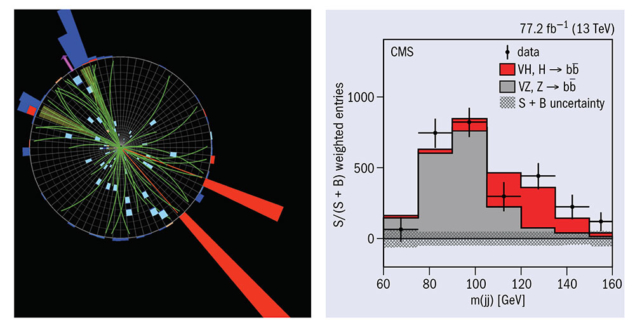
A report from the CMS experiment
The observation of the Higgs-boson decay to bottom quark–antiquark (bb̅) by the CMS experiment is a seminal achievement that sheds light on one of the key missing pieces of the Higgs sector of the Standard Model (SM).
Processes that include the Higgs boson’s favoured decay mode to b-quarks (with about 58% probability) have until now remained elusive because of the overwhelming background of b-quark events produced via strong interactions. While the recent CMS observation of Higgs-boson production in association with top quarks (ttH) constitutes the first confirmation of the tree-level coupling of the Higgs boson to quarks (CERN Courier June 2018 p10), the Higgs-boson decay to bb̅ tests directly its coupling to down-type quarks. Moreover, this decay is crucial for constraining, under fairly general assumptions, the overall Higgs-boson decay width and thus reducing the uncertainty on the measurement of absolute couplings. This observation effectively narrows down the remaining window available for exotic or undetected decays.
At the LHC, the most effective strategy to observe the Higgs bb̅ decay is to exploit the associated production mechanism with an electroweak vector boson VH, where V corresponds to a W or Z boson. The leptons and neutrinos arising from the V decay provide large suppression of the multijet background, and further background reduction is achieved by requiring the Higgs-boson candidates to have large transverse momentum.
Advanced machine-learning techniques (deep neural networks, DNN) are used in different steps of the analysis including: the b-jet identification, the measurement of the b-jet energy, the classification of different backgrounds in control regions, and the final signal extraction.
This result uses LHC data collected in 2016 and 2017 at an energy of 13 TeV and has benefited from the recent CMS pixel tracker upgrade with further improved b-quark identification performance.
A signal region enriched in VH events is selected together with several dedicated control regions to monitor the different background processes. Then, a simultaneous binned-likelihood fit of the signal and control regions is performed to extract the Higgs-boson signal.
The score of the DNN separating signal from the background is used for the signal extraction fit. Several observables are combined and the most discriminating are: the angular separation between the two b-quarks and the b-tagging properties of the Higgs candidate jets. An event candidate for the production of a Z boson in conjunction with a Higgs boson is shown in the left figure.
A clear excess of events is observed in the combined 2016 and 2017 data, in comparison with the expectation in the absence of a H → bb̅ signal. The significance of this excess is 4.4σ, where the expectation from SM Higgs-boson production is 4.2σ. The signal strength corresponding to this excess, in relation to the SM expectation, is 1.06 ± 0.26. When combined with the measurement from LHC Run 1 at 7 and 8 TeV, the signal significance increases to 4.8σ, while 4.9σ is expected. The corresponding signal strength is 1.01 ± 0.22.
The dijet invariant mass distribution (figure, right) allows for a more direct visualisation of the Higgs-boson signal. The contributions of the VH and VZ processes are separately visible, after all other background processes have been subtracted.
The VH results are combined with CMS measurements in other production processes, including gluon-fusion, vector-boson fusion, and associated production with top quarks, with data collected at 7, 8 and 13 TeV, depending on the process. The observed combined significance is raised to 5.6σ, where the expectation from SM Higgs-boson production is 5.5σ. The signal strength corresponding to this excess, relative to the SM expectation, is 1.04 ± 0.20, in perfect agreement with the latter.
With the direct observation of the Higgs-boson couplings to bottom quarks complementing those involving tau leptons and top quarks (see further reading), the Yukawa couplings to all accessible third-generation fermions have now been firmly established. This opens a new era of precision studies in the Higgs sector that will fully benefit from the larger dataset that will be available by the end of Run 2.
Further reading
CMS Collaboration 2018 Phys. Rev. Lett. 121 121801.
CMS Collaboration 2018 Phys. Lett. B 779 283.
CMS Collaboration 2018 Phys. Rev. Lett. 120 231801.