Researchers at the National Superconducting Cyclotron Laboratory (NSCL) at Michigan State University have produced the heaviest silicon isotope ever observed. The recent identification of 44Si expands the chart of known isotopes and lays the groundwork for the future study of rare, neutron-rich nuclei.
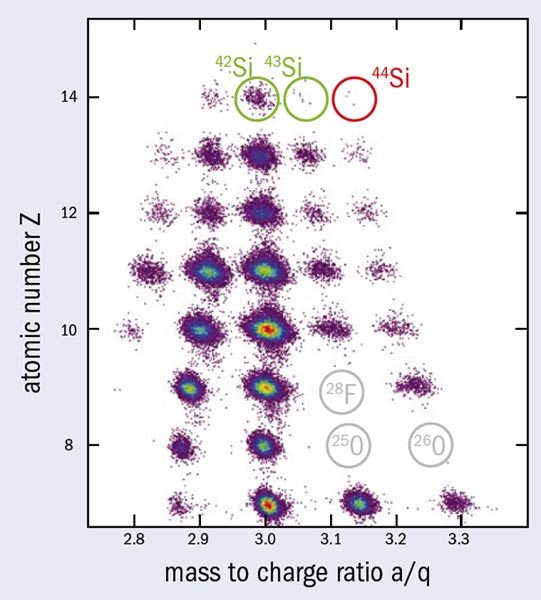
Beyond a certain range of combinations of protons and neutrons, nuclei cannot form at all, and additional nucleons will immediately leave the nucleus owing to zero binding energies. Pursuit of this limit, known as the drip line, has proved to be a scientific and technical challenge – particularly when it comes to neutron-rich nuclei. While the proton drip line has been mapped out for much of the chart of nuclei, the neutron drip line is known only up to oxygen (Z = 8). Producing isotopes at or near the neutron drip line remains a long-standing goal in experimental nuclear physics. For example, 43Si was detected for the first time at Japan’s Institute of Physical and Chemical Research (RIKEN) in 2002 (Notani et al. 2002). That same year, researchers at the GANIL laboratory in France detected the neutron-rich isotopes 34Ne and 37Na (Lukyanov et al. 2002).
In the 44Si experiment conducted at the NSCL Coupled Cyclotron Facility in January, a primary beam of 48Ca was accelerated to 142 MeV/u and directed at a tungsten target. Downstream from the target, the beam was filtered through NSCL’s A1900 fragment separator. Eventually, some 20 different isotopes (including three nuclei of 44Si) hit a set of detectors that could identify each ion as it arrived (Tarasov et al. 2007).
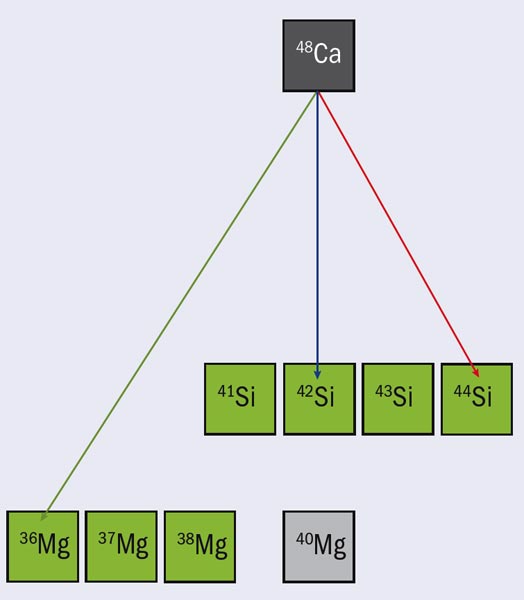
The study was intended to document the yield of isotopes containing 28 neutrons that lie between 48Ca (the nuclei in the beam) and 40Mg to extrapolate the expected yields in this region. 40Mg is yet to be observed, and according to some theories should be on the drip line. Knocking out only protons from 48Ca could create these isotopes, although this is a difficult feat because of the larger number of neutrons in the beam nuclei. The production of 44Si is therefore an even greater feat, given that the collision must also transfer two neutrons from the tungsten target to the beam nucleus as it speeds past. The observation of 44Si in the A1900 fragment separator stretches the limits of its single-stage separation. The excessive number of particles that come along with the rare nuclei can swamp the detectors used to identify the beam in the separator. The next-generation technique will use two-stage separation, delivering fewer particles to the detectors as more are filtered out travelling down the beamline.
Researchers are developing new two-stage separators that could run experiments with higher initial beam intensities, which offer a better chance of generating the sought-after, near-dripline nuclei. Preliminary testing on a new two-stage separator at NSCL has delivered promising results. Also, a new device has just been constructed at RIKEN in Japan, and one is planned for GSI in Germany. Nuclear scientists at NSCL hope that two-stage separation will help uncover the next generation of rare isotopes.
Further reading
S Lukyanov et al. 2002 J. Phys. G 28 L41.
M Notani et al. 2002 Phys. Lett. B 542 49.
O Tarasov et al. 2007 Phys. Rev. C 75 064613 and arxiv.org/abs/0705.0349.