It’s taken a while, but the world’s highest-energy accelerator is back. Fermilab’s Tevatron proton-antiproton collider is once again exploring the high-energy frontier, with newly upgraded detectors and a physics programme that will address some of the biggest questions in particle physics.

Tevatron Run II is under way at Fermilab, with upgraded detectors addressing some of the most important questions in particle physics. What is the structure and what are the symmetries of space-time? Why is the weak force weak? What is cosmic dark matter? Why is matter-antimatter symmetry not exact? Until CERN’s Large Hadron Collider (LHC) turns on, the Tevatron is the world’s only source of top quarks. It is the only place where we can directly search for supersymmetry, for the Higgs boson, and for signatures of additional dimensions of space-time. And it is also the most likely place to directly observe something totally unexpected.
After a somewhat frustrating year, recent progress with the accelerator has been gratifying. Records are regularly being broken for peak and weekly luminosities. The complex is now performing well in excess of its Run I (1992-1995) bests, and is delivering increased energy (1.96 TeV). These improvements have come from well understood modifications, and there is a detailed plan for the next steps. As Steve Holmes, Fermilab’s associate director for accelerators, has said: “There is no silver bullet to be found.” Rather, we have to make a large number of 10-15% improvements; Holmes notes that (1.15)10 = 4. The major areas to be tackled are: transfer and acceleration efficiencies; emittance dilution; beam lifetimes in the Tevatron before acceleration; and the role of long-range interactions between the beams. Significant help from other laboratories and from physicists in other parts of Fermilab has also been an important contribution. Fermilab and CERN have set up an exchange programme; Frank Schmidt and Frank Zimmerman from CERN are helping Run II to become a success, and in the future, Fermilab will send machine physicists to CERN to help with LHC commissioning.
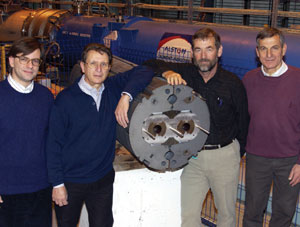
The CDF and D0 detectors are both working well, emphasizing data-taking efficiency, and are recording physics-quality data. Performance of the tracking, calorimeter and muon detectors is good, and beam-induced backgrounds are under control. CDF is now running a trigger for B-mesons using displaced tracks from the silicon detector. This is a first at a hadron collider, and has already yielded some very impressive heavy flavour samples. Processing the huge quantities of data from modern experiments is a challenge in itself, as is making it available to the large and widely distributed collaborations. There is a natural synergy between these challenges and current ideas about “Grid” computing. Both CDF and D0 are already making something like a Grid a reality, using a Fermilab-developed data distribution system called SAM to send their data out to their collaborators. They are also exploring ways for remote physicists to assist in monitoring detector operations.
Physics in Run II
The physics goals of Run II involve direct searches for as yet unknown particles and forces, including both those that are predicted or expected (like the Higgs boson and supersymmetry) and those that would come as a surprise. At the same time, we confront the Standard Model through precise measurements of the strong interaction, the quark-mixing matrix, and the electroweak force and properties of the W boson, the Z boson and the top quark. The experiments already have first results in all of these areas. Given the amount of data collected so far in Run II, they are not yet competitive with the results already published from Run I or the experiments at CERN’s LEP electron-positron collider, but they show that all the analysis tools are in place and ready.
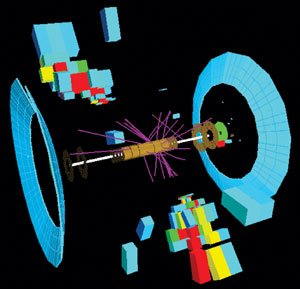
As the world’s highest-energy collider, the Tevatron is the most likely place to directly discover a new particle or force. We know the Standard Model is incomplete; theoretically the most popular extension is to make it a part of a larger picture called supersymmetry (which is a basic prediction of superstring models). Here each known particle has a so-far unobserved and more massive partner, to which it is related through a change of spin. If it exists, the lightest supersymmetric particle would be stable, and vast numbers of them would pervade the universe, explaining the astronomers’ observations of dark matter. The Tevatron is the only place now available to directly search for supersymmetry. In Run II, the opportunities for discovery include squarks and gluinos, in final states with missing energy (ETmiss) and jets (and lepton(s)); charginos and neutralinos through multilepton final states; gauge-mediated SUSY in ETmiss + photon(s) channels; stop and sbottom; and R-parity violating models. Searches for other new phenomena include leptoquarks, dijet resonances, new heavy W´ and Z´ bosons, massive stable particles, and monopoles.
The Tevatron allows us to experimentally test the new and exciting idea that gravity may propagate in more than four dimensions of space-time. If there are extra dimensions that are open to gravity, but not to the other particles and forces of the Standard Model, then we could not perceive them in our everyday lives. However, particle physics experiments at the TeV scale could see signatures such as a quark or gluon jet recoiling against a graviton, or indirect indications like an increase in high-energy electron-pair production. These studies use the Tevatron to literally measure the shape and structure of space-time.
While it is good to be guided by theory, one should also remain open to the unexpected. Therefore both experiments have developed quasi-model-independent (signature-based) searches, which look for significant deviations from the Standard Model. In the Run I dataset, no significant evidence for new physics was found. Perhaps revealing different psychologies, D0 has quantified its agreement with the Standard Model at the 89% confidence level, while CDF has preferred to highlight some potential anomalies as worth pursuing early in Run II.
The experiments have already embarked on a number of searches using Run II data. Work has started on understanding the ETmiss distribution in multijet events as a prelude to squark and gluino searches; trilepton candidates are also being accumulated. At D0, a gauge-mediated SUSY search has set a limit on the cross section for pp-Æ ETmiss + gg. Also at D0, virtual effects of extra dimensions are being sought in e+e–, m+m– and gg final states, and limits on the scale of new dimensions at the 0.9 TeV level can already be set. A search for leptoquarks decaying to electron + jet has been carried out. So far, none of the cross sections or mass limits is better than published Run I results, but it serves as a demonstration that the pieces are all in place.
In the Standard Model, the weak force is weak because the W and Z bosons interact with a field (the Higgs field) that permeates the universe. This same field gives masses to all the fundamental fermions. It should be possible to excite this field and observe its quanta – the long sought-after Higgs boson. It is the last piece of the Standard Model, and also the key to understanding any beyond-the-Standard-Model physics like supersymmetry. Finding it is a very high priority. Right now, we are developing the foundations needed for Higgs physics in Run II: good jet resolution; high b-tagging and trigger efficiencies; and a good understanding of all the backgrounds. One area that can be attacked with relatively modest luminosities in 2003 is to search for one or more of the extended suite of Higgs bosons that are predicted in supersymmetric models. Associated production of a SUSY Higgs together with a bb- pair is enhanced at high tanb, and we will be able to improve on present limits with only a few hundred inverse picobarns.

In Run II, we will complement our direct searches for new phenomena with indirect probes. New particles and forces can be seen indirectly through their effects on electroweak observables. The tightest constraints will come from improved determination of the masses of the W boson and the top quark. Both experiments now have preliminary results from their Run II samples of W and Z candidates. They have measured the cross sections at the Tevatron’s new centre of mass energy of 1.96 TeV and used the ratio of the W to the Z to indirectly extract the W width. CDF has also taken a first look at the forward-backward asymmetry in e+e– production in Run II. Currently, the W mass is known to be mW = 80 451 ± 33 MeV; the measurement is dominated by LEP data. Our Run I results fixed the W mass at the 60 MeV level, but it will take a Run II dataset of order 1 fb-1 before we can significantly improve the world knowledge of mW – not a short-term prospect. Given 2 fb-1 we will be able to drive the uncertainty down to the 25 MeV level per experiment, with an ultimate capability of 15 MeV per experiment.
The Tevatron collider is the world’s only source of top quarks. The top quark was discovered by CDF and D0 in 1995 on the basis of a few tens of events – they are now gearing up to study top quarks in the thousands. The top is the heaviest known quark and, alone among quarks, couples strongly to the Higgs. We need to test its properties and decays with sufficient precision to confirm the Standard Model – is the top really top? Here we can look forward to significant improvements in the short term, because the Run I dataset was so statistically limited. Both D0 and CDF are on the road to “rediscovering” top for the spring 2003 conferences, and both experiments have candidate events. Per inverse femtobarn, we will collect roughly 500 b-tagged top-pair events in the lepton + jets final state. As well as improving the cross-section and mass measurements, we will look for top-antitop spin correlations which can tell us if the top is really the spin-1/2 object it should be, and observe single top production (which allows a model-independent measurement of the Cabibbo-Kobayashi-Maskawa (CKM) quark mixing matrix element |Vtb|). New techniques are also being developed: D0 has a new, preliminary determination of the top mass from Run I data that uses more information per event, obtains a better discrimination between signal and background than the published 1998 analysis, and improves the statistical error equivalently to a factor 2.4 increase in the number of events. Run II will also test beyond-the-Standard-Model theories that predict unusual top properties, states decaying into top, and anomalously enhanced single top production.
The mixing between the three generations of quarks results in subtle violations of the so-called CP symmetry relating particles and antiparticles. Understanding this symmetry will help to explain why the universe is filled with matter, not antimatter. In the decays of B-mesons, these symmetry violations can be large, and so B-hadrons have become an important laboratory to explore the “unitarity triangle”, which relates the elements of the CKM quark-mixing matrix. In Run II, we want to confront the CKM matrix in ways that are complementary to the electron-positron B-factories. CP violation is now established in the B system through the decay Bd Æ J/y Ks. The measured mixing angle is consistent with the Standard Model, but cannot exclude new physics by itself. The BaBar and BELLE experiments can and will do much more with their data, but the Tevatron can uniquely access the Bs meson, which is not produced at the B-factories, and has therefore been called the “El Dorado” for hadron collider B-physics. By measuring the mixing rate between Bs and B-s, we can determine the length of one of the sides of the unitarity triangle and complement the B-factories’ measurements of its angles. CDF expects to be sensitive to Standard Model mixing with a few hundred inverse picobarns. It will also be interesting to see if there is sizeable CP violation in Bs Æ J/y f (it is expected to be small); while the decay Bs Æ KK at the Tevatron complements B d Æ pp that is measured at the B-factories. Together they can pin down the triangle angle g. There are many other opportunities, such as Lb properties and searches for rare decays. CDF already has most impressive results from Run II, building on its Run I experience together with new detector capabilities (silicon vertex trigger and time-of-flight detector). Lepton-triggered signals for B± Æ J/y K±, B0 Æ J/y K*0 and Bs Æ J/y f are seen, while using the silicon vertex trigger, the purely hadronic modes B± Æ D0p Æ Kpp and B Æ hadron hadron are being recorded. We can also look forward to CDF exploiting an enormous sample of charm mesons. In D0, the tools are being put in place for a B-physics programme. The inclusive B lifetime has been measured, and B-mesons are being reconstructed. D0 does not exploit purely hadronic triggers, but benefits from its large muon acceptance, forward tracking coverage, and ability to exploit J/y Æ e+e–.
No one doubts that quantum chromodynamics (QCD) describes the strong interaction between quarks and gluons. Its effects are all around us – it is the origin of the masses of hadrons, and thus of the mass of stars and planets. This doesn’t mean it is an easy theory to work with. As well as using hadron colliders to test QCD itself, we find that it is so central to the calculation of both signal and background processes that we need to make sure we can have confidence in our ability to make predictions in this framework. We need to resolve some outstanding puzzles and ensure we understand how to calculate the backgrounds to new physics.
Both CDF and D0 have now measured jet-energy distributions from Run II. CDF are making use of their new forward calorimetry to cover the whole range of pseudorapidity. Jet calibrations are not yet final, but already we see events with transverse energies beyond 400 GeV. With the full Run II dataset this will reach as far as 600 GeV, allowing us to pin down the high-energy behaviour of the cross section, and thus the gluon content of the proton (which remains poorly determined at high momentum and a source of uncertainty). Another issue provoking much discussion is the choice of the algorithm used to define jets. D0’s Run I data have shown that the two most popular jet definitions (the geometrically based “cone” and the momentum-based recombination “k^” algorithms) yield different cross sections for collider data; while qualitatively as expected, quantitatively it is not yet clear whether the differences are understood. We will try to address this question with early Run II data.
Run I left many unanswered questions about heavy flavour (charm and bottom) production. Resolving these is important, because many new particles result in heavy flavour signatures. The inclusive B-meson production cross section lies significantly above the QCD prediction, though it can be made to fit better using resummation and retuned fragmentation functions (from LEP data). For charmonium, the measured cross section requires a large colour-octet component, but that is not consistent with the observed J/y polarization. The CDF secondary vertex trigger in Run II is working beautifully, and the resulting huge charm and bottom samples will allow these puzzles to be explored in much more detail. D0 now has preliminary Run II J/y and muon + jet cross sections which are the first steps in measuring the charmonium polarization (and thus production process) and the b-jet cross section.
Another QCD-related puzzle is hard diffraction. In these events, a high-momentum-transfer collision occurs, but one of the incoming beam particles appears to leave the collision intact, instead of being destroyed in the process. This observation is rather surprising and needs to be pinned down better and related quantitatively with similar phenomena observed at HERA. Both CDF and D0 have new instrumentation for diffractive physics in Run II. This will allow us to test some of the basic assumptions that have gone into earlier studies, and will provide a sanity check for ideas of Higgs production through this mechanism at the LHC.
Planning for the future

At the same time as the Fermilab accelerator experts have been working to improve Tevatron operations, they have been trying to incorporate the lessons learned into a solid plan for the future. The planning for the accelerator complex is in two phases. The first focuses on US fiscal year 2003, which ends in September 2003. A full plan and schedule are in place. The US Department of Energy (DOE) recently convened a high-level international review committee, chaired by David Sutter of the DOE, to look at this plan. The committee complimented the laboratory on its recent luminosity progress and its focus on the colliding beam programme, and reported that the goals for luminosity in 2003 are highly likely to be met. By summer 2003, each experiment should have recorded around 200 pb-1 of Run II data (almost twice the Run I dataset). The centrepiece will be a greatly increased top quark sample, thanks to the higher beam energy and the much improved b-tagging capabilities of the detectors. A first look at Bs mixing will be possible, together with lifetimes and branching ratio measurements from the B, Bs, Lb and charm samples. Jet distributions at the highest energies will constrain proton structure, and searches will follow up on Run I anomalies and extend the Run I reach for many extensions to the Standard Model.
The second phase covers 2004 and beyond. It is now clear that it will take somewhat longer than had been anticipated to accumulate the large datasets ultimately foreseen for Run II: such is the price of realism. As long as the Tevatron remains the world’s highest-energy collider, it is a unique facility that must be exploited to its limit; this will remain true until the LHC experiments start producing competitive physics results. We are preparing to run the Tevatron until the end of the decade in order to fully realise its physics potential. The Run II physics programme is a broad and deep one, and will answer crucial questions about the universe. There is no threshold at which this starts (figure 1). There is compelling physics to be done each year and with each doubling of luminosity, starting now with a few hundred inverse picobarns, and to the end of the decade with multifemtobarn data sets. To explore the 5-15 fb-1 domain calls for upgrades to the CDF and D0 detectors. Primarily, these involve new trigger systems to handle more than 10 interactions per crossing at the expected luminosity, and new silicon detectors that make use of LHC R&D to sustain the high radiation doses. These upgrades were successfully reviewed by the DOE in September, and are now moving towards approval, with installation planned for 200502006.
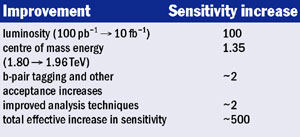
In summary, Run II at the Tevatron provides extraordinary opportunities for the advancement of our knowledge of particle physics. A measure of the increased sensitivity, using top quark production as an example, is shown in Table 1. With a factor 500 increase in sensitivity, the CDF and D0 collaborations are eager to thoroughly explore the energy frontier before passing the baton to the ATLAS and CMS experiments at the LHC.
As Jay Marx from Lawrence Berkeley National Laboratory pointed out at the accelerator review, Run II is a marathon and not a sprint. The combination of high accelerator energy, excellent detectors, enthusiastic collaborations and data samples that are doubling every year guarantees interesting new physics results at each step. Each step answers important questions, and each leads on to the next. This is how we will lay the foundations for a successful LHC physics programme – and hopefully a linear collider to follow.