Christian Fabjan looks at the challenges that the experiments faced.
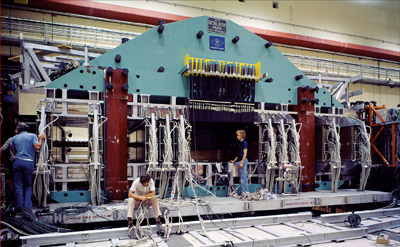
The committee for the ISR experimental programme – the ISRC – started its work in early 1969, with the collider start-up planned for mid-1971. Two major lines of experimental programmes emerged: “survey experiments” would aim to understand known features (in effect, the Standard Model of the time) in the new energy regime, while other experiments would aim at discoveries. This was surprisingly similar to the strategy 40 years later for the LHC. But in reality the two approaches are worlds apart.
Hadronic physics in the late 1960s was couched in terms of thermodynamical models and Regge poles. The elements of today’s Standard Model were just starting to take shape; the intermediate vector bosons (W+, W– and W0, as the Z0 was called then) were thought to have masses in the range of a few giga-electron-volts. The incipient revolution that was to establish the Standard Model was accompanied by another revolution in experimentation. Georges Charpak and collaborators had demonstrated the concept of the multiwire proportional chamber (MWPC) just one year earlier, propelling the community from a photographic-analogue into the digital age with his stroke of genius. Nor should the sociological factor be forgotten: small groups, beam exposures of a few days to a few weeks, as well as quick and easy access to the experimental apparatuses – all characterized the style of experimentation of the time.
These three elements – limited physics understanding, collaboration sociology and old and new experimental methods – put their stamp on the early programme. From today’s perspective, particle physics was at the dawn of a “New Age”. I will show how experimentation at the ISR contributed to the “New Enlightenment”.
1971–1974: the ‘brilliant, early phase’
Maurice Jacob, arguably one of the most influential guiding lights of the ISR programme, called this first period the “brilliant, early phase”, in reference to its rich physics harvest (Jacob 1983). The lasting contributions include: the observation of the rising total-cross section; measurements of elastic scattering; inclusive particle production and evidence for scaling; observation of high-pT production; and the non-observation of free quarks. Several experimental issues of the period deserve particular mention.
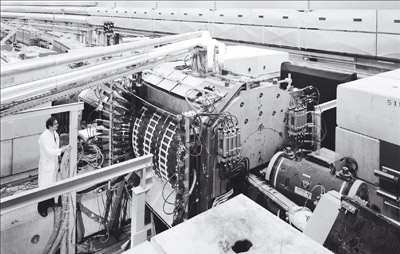
The experimental approach matched the “survey” character of this first period. The ISR machine allowed tempting flexibility, with operation at three – later four – collider and asymmetric beam energies. Requests for low- or high-luminosity running and special beam conditions could all be accommodated. A rapid switch-over from one experimental set-up to another at the same interaction point was also one of the guiding design principles. Notwithstanding this survey character, this early period saw several imaginative contributions to experimentation – some with a lasting influence.
The devices known today as “Roman pots” were invented at the ISR with the aim to place detectors close to the circulating beams, which was a requirement for elastic-scattering experiments in the Coulomb interference region. During beam injection and set up these detectors had to be protected from high radiation doses by retracting them into a “stand-by” position. The CERN-Rome group (R601, for experiment 1 at intersection 6 (I6)) in collaboration with the world-famous ISR Vacuum group developed the solution: the detectors were housed in “pots” made from thin stainless-steel sheets, which could be remotely moved into stand-by mode or one of several operating positions. This technique has been used at every hadron collider since, including at the LHC.
The first 4π-detector was installed by the R801 Pisa-Stony-Brook collaboration. It used elaborate scintillator hodoscopes, providing 4π-coverage with high azimuthal and polar-angle granularity, well adapted to the measurement of the rising total cross-section and short-range particle correlations. The Split-Field Magnet (SFM), ultimately installed at interaction 4 (I4), was the first general ISR facility. The SFM was groundbreaking in many ways and was proposed by Jack Steinberger in 1969 as the strategy for exploring terra incognita at the ISR with an almost 4π magnetic facility.
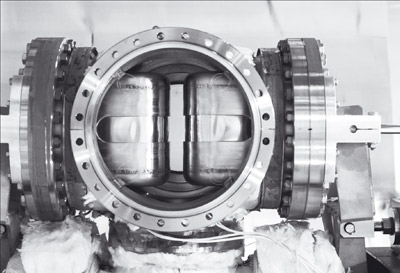
The SFM’s unconventional magnet topology – two dipole magnets of opposite polarity – addressed dual issues: minimizing the magnetic interference with the two ISR proton beams; and providing magnetic analysis preferentially in the forward region, the place of physics interest according to prevailing understanding. It made daring use of the new MWPC technology for tracking, propelling this technique within a few years from 10×10 cm2 prototypes to instrumentation of hundreds of MWPCs with hundreds of square metres and almost 100,000 electronic read-out channels. The SFM became operational towards the end of 1973 – a fine example of what CERN can accomplish with a meeting of minds and the right leadership. True to its mission this facility was used by many collaborations, changing the detector configuration or adding detection equipment as necessary, throughout the whole life of the ISR. The usefulness of a dipole magnetic field at a hadron collider was later to be beautifully vindicated by the magnetic spectrometer of the UA1 experiment in the 1980s.
The Impactometer was the name given by Bill Willis to a visionary 4π detector, proposed in 1972 (Willis 1972). It anticipated many physics issues and detection features that would become “household” concepts in later years. The 4π-coverage, complete particle measurements and high-rate capabilities were emphasized as the road to new physics. One novel feature was high-quality electromagnetic and hadronic calorimetry, thanks to a futuristic concept: liquid-argon calorimetry. In a similar spirit, an Aachen-CERN-Harvard-Genoa collaboration, with Carlo Rubbia and others, proposed a 4π-detector using total-absorption calorimetry but based on more conventional techniques: among the three options evaluated were iron/scintillator, water Cherenkovs and liquid-scintillator instrumentation. However, the ISRC swiftly disposed of both this proposal and the Impactometer.

The discovery of high-pT π0-production at rates much higher than anticipated was one of the most significant early discoveries at the ISR, which profoundly advanced the understanding of strong interactions. Unfortunately, this physics “sensation” proved also to be an unexpected, ferocious background to other new physics. Discovered by the CERN-Columbia-Rockefeller (CCR, R103) collaboration in 1972, the high rate of π0s masked the search for electrons in the region of a few giga-electron-volts as a possible signal for new physics – as in, for example, the decay of an intermediate vector boson into e+e– pairs – and ultimately prevented this collaboration from discovering the J/ψ.
The reaction to this “sensation plus dilemma” was immediate, resulting in several proposals for experiments, all of which were capable of discoveries – as their later results demonstrated. These more evolved experimental approaches brought a new level of complexity and longer lead-times from proposal to data-taking. However, the fruition of these efforts came a few years too late to make the potentially grand impact that was expected from and deserved by the ISR.
In 1973, the CCOR collaboration (CCR plus Oxford) proposed the use of a superconducting solenoid, equipped with cylindrical drift chambers for tracking and lead-glass walls for photon and electron measurements (R108). The Frascati-Harvard-MIT-Naples-Pisa collaboration proposed an instrumented magnetized iron toroid for muon-pair studies and associated hadrons. Originally intended for installation in I8 (R804), it was finally installed because of scheduling reasons in I2 (R209). The SFM facility was complemented with instrumentation (Cherenkov counters and electromagnetic calorimetry) for electron studies, and later for charm and beauty studies.

The 1972 Impactometer proposal was followed with a reduced-scale modular proposal concentrating on e+e– and γ-detection, submitted in November 1973 by a collaboration of Brookhaven, CERN, Saclay, Syracuse and Yale. It combined the liquid-argon technology for electromagnetic calorimetry with novel transition-radiation detectors for electron/hadron discrimination. (These latter consisted of lithium-foil radiators and xenon-filled MWPCs, with two-dimensional read-out, as the X-ray detectors.) The advanced technologies proposed led to the cautious approval of the detector as R806T (T for test) in June 1974 with a gradual, less than optimal build-up.
1974-1977: learning the lessons
The first “brilliant period” ended with a Clarion call for the particle-physics community at large and sobering soul-searching for the ISR teams: the discovery of the J/ψ in November 1974. The subsequent period brought a flurry of activities, with the initial priority to rediscover the J/ψ at the ISR.
First was R105 (CCR plus Saclay), which employed threshold Cherenkovs and electromagnetic calorimeters, permitting electron identification at the trigger level. Second, an overwhelmingly clear physics justification for a new magnetic facility with an emphasis on high-pT phenomena, including lepton detection, emerged. Several groups, including teams from the UK and Scandinavia, were studying a facility based on a large superconducting solenoid, while a team around Antonino Zichichi explored the potential of a toroidal magnetic facility. The inevitable working group, constituted by the ISRC and chaired by Zichichi, received the remit to motivate and conceptualize a possible new magnetic facility.
With exemplary speed – January to March 1976 – the working group documented the physics case and explored magnets and instrumentation, but shied away from making a recommendation, leaving the choice between toroid and solenoid to other committees. It is a tribute to the ISRC that it made a clear recommendation for a solenoid facility with large, open structures in the return yoke for additional instrumentation (particle identification and calorimetry). The toroidal magnet geometry, while recognized as an attractively suitable magnet topology for proton–proton collider physics, was considered too experimental a concept for rapid realization. It would take another 30 years before a major toroid magnet would be built for particle physics, namely the ATLAS Muon Spectrometer Toroid.
The CERN Research Board did not endorse the ISRC recommendation, possibly being concerned – I am speculating – about the constraints on the long-term impact on the ISR schedule and adequate support among the user community. Despite this negative outcome, the working group had a significant influence on CERN’s research agenda. It provided an assessment of state-of-the-art collider experimentation and many members of the working group would use their work to shape the UA1 and UA2 facilities at the SppS programme, which was proposed at about the same time.
Within weeks following the negative decision from the Research Board, some key members of the working group banded together and submitted a new proposal for a fully instrumented facility, building around Tom Taylor’s innovative Open Axial Field Magnet (warm Helmholtz coils with conical poles), as a base for the Axial Field Spectrometer (AFS). The time was just right: it took only three months from the submission of the proposal by the CERN-Copenhagen-Lund collaboration in January 1977 to ISRC recommendation and Research Board approval as R807 in April, thanks to the strong and courageous support from the then Research Director, Paul Falk-Vairant, and the committees.

The end of this period also coincided with a turning point in our understanding of hadronic interactions. The early view of “soft” hadronic interactions, limited to low-pT phenomena, shaped the initial programme. Ten years later, hadrons were still complicated objects but the point-like substructure had been ascertained. Hard scattering became the new probe and simplicity was found at large pT with jets, photons and leptons. This marked a remarkable “about-turn” in our approach towards hadron physics, which found its expression in the second half of the ISR experimentation and exploitation.
1977–1983: maturity
The shock of 1974, followed by the debates on physics and detector facilities in 1976 focused the minds of the various players. Experimental programmes were being prepared for (relatively) rare, high-pT phenomena, in a variety of manifestations: leptons, photons, charmed particles, intermediate vector bosons with a sensitivity reaching beyond 30 GeV/c2 and jets. This strategy was vindicated by the discovery at Fermilab of the Υ in July 1977. This was yet another cruel blow to the ISR, particularly considering that the R806 collaboration saw the first evidence for the Υ at the ISR in November 1977 (Cobb et al. 1977).
The versatility of the ISR and the incipient SppS development brought also proton–antiproton collisions and light-ion (d, α) physics to the fore. A multifaceted and promising programme – confirmed by the 2nd ISR Physics Workshop in September 1977 – was being put in place. By early 1978 the efforts that were started in 1973 and 1974 brought their first fruits: the R108 collaboration reported their first results at the 1978 Tokyo Conference; the R209 collaboration had their experiment completed by the end of 1977; R806 was completed by early 1976; and R807 was building up to a first complete configuration towards the end of 1979. A walk round the ISR-ring would have shown the diversity of approaches that were being adopted:
• I1 was home for the R108 collaboration using an advanced, thin (1 radiation length) 1.5 T superconducting solenoid, which would become its workhorse for the subsequent six years. This was instrumented with novel – at the time – cylindrical drift chambers inside and lead-glass electromagnetic-shower detectors outside. Several upgrades brought higher sensitivity through the addition of shower counters inside the solenoid, which resulted in full azimuthal coverage both for charged particles and for photons, as well as higher collision rates, as provided by the inventive ISR teams in the form of warm, low-beta quadrupoles for stronger beam focusing (p27).
• I2 was truly complementary to I1, with the R209 (CERN-Frascati-Harvard-MIT-Napoli-Pisa) collaboration betting on muons and magnetized steel toroids and aiming at dimuon mass sensitivity beyond 30 GeV/c2. This was combined with a large-acceptance hadron detector, based on scintillator hodoscopes for hadron correlation studies.
• I4 was where the SFM showed its strength as a “user facility”, accommodating the 22nd ISR experiment, R422, at the end of the ISR. The open magnet structure invited many groups to add equipment for dedicated low- to high-pT physics, with remarkable contributions to charm physics and candidates for Λb.
• I6 explored physics in the forward region with an unusual magnet, known as the “Lamp Shade”. It also had considerable emphasis on charm particles.
• I7 was reserved for “exotica”. In the late 1970s, a group operated a streamer chamber as a rehearsal for what later would become UA5 at the SppS. The last experiment to take data – after the official closure of the ISR on 23 December 1983 – was R704, which used 3.75 GeV/c antiprotons colliding with an internal gas-jet of H2 to perform charmonium spectroscopy.
• I8 became the home of R806, with its finest hour being the discovery of prompt γ production, the golden test-channel for perturbative QCD. It entered into a rich symbiotic relation with the nascent AFS (R807) between the end of 1979 and late 1981, when all of R807 was installed except for the uranium/scintillator calorimeter. After a considerable struggle to obtain the uranium plates, this advanced (and adventurous) hadron calorimeter was finally completed by early 1982. One of the significant results obtained with the calorimeter was the first measurement of the jet production cross-section at ISR energies in 1982, consistent with QCD predictions. With the closure of 2π calorimeter coverage, R806 finally had to yield its place, morphing into two novel photon detectors (NaI crystals with photodiode read-out). The Athens-Brookhaven-CERN-Moscow collaboration (R808) provided these detectors, which were placed on opposite walls of the uranium/scintillator calorimeter. In its final years, the ISR machine teams integrated superconducting low-beta quadrupoles, providing peak luminosities in excess of 1032 cm–2 s–1 – a superb rehearsal for the LHC.
The effect of a concept-driven revolution is to explain old things in new ways. The effect of a tool-driven revolution is to discover new things that have to be explained!
Freeman Dyson
The final year, 1983, saw a valiant struggle between the physics communities, hell-bent on extracting the most physics from this unique machine – proton–proton, light ions, proton–antiproton operation with a total of almost 5000 hours of physics delivered – and a sympathetic, yet firm director-general, Herwig Schopper, who presided over the demise of the ISR. In the last session of the ISRC he not only paid tribute to the rich physics harvest but also emphasized the important and lasting contribution of the ISR to experimentation at colliders – or, in the words of one of today’s most brilliant theorists, Freeman Dyson: “New directions in science are launched by new tools more often than by new concepts. The effect of a concept-driven revolution is to explain old things in new ways. The effect of a tool-driven revolution is to discover new things that have to be explained!”
• I am grateful to M Albrow, G Belletini, L Camilleri and W Willis for discussions and careful reading.