The ERL2005 Workshop at Jefferson Lab in March – the first of its kind – reviewed an innovative use of electron linacs in light sources and, potentially, particle colliders.
In March, 159 scientists from around the world gathered at the US Department of Energy’s (DOE’s) Jefferson Lab (JLab) in Newport News, Virginia, for ERL2005, the first international workshop dedicated to energy-recovering linear accelerators (ERLs). The workshop was conceived during accelerator discussions preceding the publication in 2003(p13) of the DOE’s Facilities for the Future of Science: A Twenty-Year Outlook.
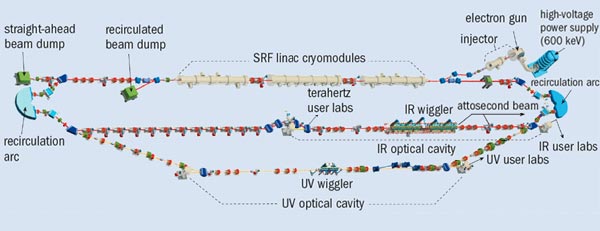
Those discussions initially focused on the need to develop high-brightness, high-current injectors, but soon expanded to include the ERLs then beginning to be implemented on three continents. Planning ensued for ERL2005, which was approved by the International Committee for Future Accelerators (ICFA) as an Advanced ICFA Beam Dynamics Workshop, and interest quickly grew. Other sponsors included three institutions building or planning to build superconducting radio-frequency (SRF) ERLs: Cornell University and Brookhaven National Laboratory in the US and the Council for the Central Laboratory of the Research Councils’ (CCLRC’s) Daresbury Laboratory in the UK.
The growth of ERLs
ERLs began to come of age in 1999 at a light source at JLab – the SRF ERL-driven free-electron laser (FEL). At present, several ERL projects around the world are under design or construction, and test facilities at several laboratories have been funded. Unlike the recycling of electrons in a synchrotron or a storage ring, an ERL uses a conceptually simple phasing technique to recycle the electrons’ energy. On a path measuring exactly an integer multiple of the linac RF wavelength plus a half-wavelength, an ERL’s accelerated beam travels through an experiment and re-enters the linac to yield back its energy, via the RF field, to the beam being accelerated. The decelerated beam is then dumped at low energy.
An obvious advantage of ERLs is economic. Consider, for example, the ERL-driven 4th Generation Light Source (4GLS) facility planned for Daresbury, where a prototype ERL is under construction. In its May 2003 issue (p7), Physics World reported that without energy recovery, “4GLS would consume roughly the output of a large commercial power station”. Energy recovery also simplifies spent-beam disposal.

The overall promise of ERLs has been distilled in a paper by JLab’s Lia Merminga, who chaired ERL2005 with Swapan Chattopadhyay, also from JLab. Together with co-authors D R Douglas and G A Krafft, Merminga wrote: “At the most fundamental level, beam-energy recovery allows the construction of electron linear accelerators that can accelerate average beam currents similar to those provided by storage rings, but with the superior beam quality typical of linacs. Such an ability to simultaneously provide both high current and high beam quality can be broadly utilized in, for example, high-average-power free-electron laser sources designed to yield unprecedented optical beam power; light sources extending the available photon brilliance beyond the limits imposed by present-day synchrotron light sources; electron cooling devices which would benefit from both high average current and good beam quality to ensure a high cooling rate of the circulating particles in a storage ring collider; or, possibly, as the electron accelerator in an electron-ion collider intended to achieve operating luminosity beyond that provided by existing, storage-ring-based colliders” (Merminga et al. 2003).
Realizing these prospects will require overcoming the technical challenges that the workshop was convened to discuss. These include polarized and unpolarized photoinjectors with high average current and low emittance; optimized lattice design and longitudinal gymnastics; beam stability and multibunch/multipass instabilities; beam-halo formation and control of beam loss; SRF optimization for continuous-wave, high-current applications; higher-order-mode (HOM) damping and efficient extraction of HOM power; RF control and stability; synchronization; and high-current diagnostics and instrumentation.
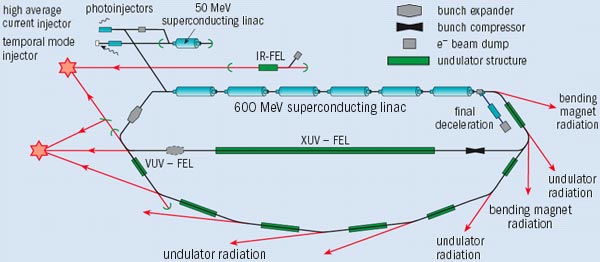
Neither the energy-recovery idea nor its close association with SRF is new. In 1965, Cornell’s Maury Tigner suggested a possible collider combining the then-novel concept of the superconducting linear accelerator with what he called “energy recovery” – an “artifice”, he wrote, that “might also be useful in experiments other than the clashing-beam type” (Tigner 1965). Energy recovery was demonstrated as early as the mid-1970s, but the first ERL with high average current drove the first kilowatt-scale FEL from 1999 to 2001 at JLab.
That FEL, which was later substantially upgraded, gave users infrared light at 3-6 μm for 1800 hours – the most achievable with available funding – and led to publications by some 30 groups. Research topics included nanotube production, hydrogen-defect dynamics in silicon, and protein energy transport. The experimentation influenced thinking about linear and nonlinear dynamical processes. Moreover, the ERL itself directly produced broadband light in the terahertz region between electronics and photonics, at over four orders of magnitude higher average power than anywhere before. In Nature, Mark Sherwin of the University of California, Santa Barbara (UCSB) predicted “new investigations and applications in a wide range of disciplines” (Sherwin 2002).

At 5 mA and 42 MeV, JLab’s original SRF ERL was a small but much-higher-current cousin of CEBAF, the five-pass, 6 GeV recirculating linac that enables the laboratory’s main mission of research in nuclear physics. The ERL/FEL has now been upgraded to produce light at 10 kW in the infrared, with a 1 kW capability imminent in the ultraviolet (figure 1). For infrared operation, the average beam current has been doubled to 10 mA. In the further evolution of ERLs, high average current will be crucial. Optimal performance, in fact, is a trade-off between that and beam degradation. Envisaged ERL projects involve average currents about an order of magnitude higher than those demonstrated so far.
In his plenary speech at the workshop, Todd I Smith of Stanford University summarized the status and outlook for ERL-based FELs. After mentioning electrostatic machines at UCSB, the College of Judea and Samaria in Israel, the Korea Atomic Energy Research Institute (KAERI) in South Korea, and FOM Nieuwegein in the Netherlands, he moved on to JLab and the other two operational RF linac FELs – an SRF machine at the Japan Atomic Energy Research Institute (JAERI) and a room-temperature ERL at the Budker Institute for Nuclear Physics (BINP), Novosibirsk. Smith said that energy-recovering RF-linac-based FELs are proliferating at a rate both “astonishing” and “satisfying”. Among those being planned are machines at KAERI, at Saclay in France and 4GLS at Daresbury. In Florida, in partnership with JLab and UCSB, the National High-Field Magnetic Laboratory has proposed initial steps toward a 60 MeV SRF ERL to drive a kilowatt FEL spanning a wavelength range of 2-1000 μm.
Let there be light
All existing hard X-ray synchrotron radiation facilities are based on storage rings. A half-century ago, first-generation synchrotron-light devices tapped particle accelerators parasitically. Then came a second generation of light sources that were based on dedicated storage rings, followed, in the 1990s, by third-generation machines with high brightness. Third-generation facilities include short-wavelength hard X-ray sources (such as the European Synchrotron Radiation Facility in Grenoble, the Advanced Photon Source at Argonne, and SPring-8 in Japan) and long-wavelength soft X-ray sources (such as the Advanced Light Source at Berkeley, the Synchrotrone Trieste in Italy, the Synchrotron Research Center in Taiwan, and the Pohang Light Source in South Korea). Fourth-generation X-ray light sources based on FELs driven by linacs are under development at DESY, SLAC and RIKEN’s Harima Institute in Japan. The idea of an X-ray synchrotron light source based on ERLs was advocated in 1998 by G Kulipanov, N Vinokurov and A N Skrinsky at BINP, with their pioneering MARS proposal, and later by JLab’s Geoffrey Krafft.
Serious pursuit of a design for an ERL light source by Cornell has recently yielded funding from the US National Science Foundation (NSF) to begin developing a major ERL-based upgrade of the Cornell High Energy Synchrotron Source at the Cornell Electron Storage Ring. ERLs also constitute “a natural and cost-effective upgrade path” for storage-ring light sources, according to Charles K Sinclair of Cornell. At the workshop, Sinclair characterized the potential improvements in ERLs in brightness, coherence and pulse brevity as “transformational”. In one of his examples of applications, he noted that on the timescale of hundreds of femtoseconds, an ERL can enable experimenters to follow the structure of ultrafast chemical reactions. With the NSF funding, Cornell is developing an injector to deliver low-emittance beams at 100 mA.
At JLab – Cornell’s partner in preparing the NSF proposal – collaborative experiments are being conducted concerning other issues in ERL development: beam break-up in the ERL/FEL and RF control in both the ERL/FEL and CEBAF. To complement the FEL’s demonstration of high average current, CEBAF was specially configured briefly during 2003 for a single-pass proof-of-principle study of energy recovery at the giga-electron-volt scale. JLab’s assets for developing SRF-driven ERLs also include the Center for Advanced Studies of Accelerators (CASA) and the Institute for SRF Science and Technology, housed in a test laboratory with a substantial complement of SRF R&D facilities.
As a first step in the 4GLS project, Daresbury is building a 50 MeV prototype ERL that will supply electron beams to a test FEL using an infrared wiggler on loan from JLab. Eventually, with a 600 MeV ERL, 4GLS would complement the UK’s higher-energy X-ray light source, Diamond, which is under construction at the CCLRC’s Rutherford Appleton Laboratory. The 4GLS facility is planned to exploit the sub-picosecond regime and to combine exceptionally high transverse and longitudinal brightness. Central to the plan are a variety of opportunities for pump-probe experiments and the combining of spontaneous and stimulated sources at a single centre. Two photocathode guns are planned, one for high average current, the other for high peak current (figure 2).
For physics research conducted at colliders, ERLs offer the promise of providing electron cooling for hadron storage rings and high-current, low-emittance electron beams for high-luminosity electron-ion colliders. In the cooling process, which brings higher luminosity to ion-beam collisions, an ion beam and an electron beam are merged. The electron beam’s energy is chosen to match the ion beam’s velocity, enabling the electron beam to remove thermal energy from the ion beam. An R&D ERL designed for 0.5 A average current is under construction at Brookhaven. It serves as a prototype for the electron cooler designed for RHICII, the proposed upgrade that could increase the luminosity of the Relativistic Heavy Ion Collider (RHIC) by an order of magnitude. It is also a prototype for an envisaged RHIC upgrade called eRHIC, in which an ERL would provide electron beams for electron-ion collisions. A similar concept, ELIC, envisages the upgrade of CEBAF at JLab for energy-recovering acceleration of electrons to use in collisions with light ions from an electron-cooled ion-storage ring.
A new ERL/FEL concept known as the “push-pull FEL” was presented at ERL2005 by Andrew Hutton of JLab. This proposal, which in some ways resembles a high-energy collider configuration mentioned in Tigner’s 1965 paper, calls for two sets of superconducting cavities with two identical electron beams travelling in opposite directions. Each set of cavities accelerates one electron beam and decelerates the other. This arrangement allows the energy used to accelerate one beam to be recovered and used again for the other. The difference compared with other energy-recovery proposals is that each electron beam is decelerated by a different structure from the one that accelerated it, so this is energy exchange rather than energy recovery. The push-pull approach can lead to a compact layout (figure 3).
The continued success of ERLs would most likely accelerate interest in Chattopadhyay’s call for “practical, affordable yet unique and exciting new accelerator facilities” at the “mezzo scale”. Such successes would also, as Merminga and colleagues concluded, “set the stage for high-energy machines at the gigawatt scale, providing intense, high-quality beams for investigation of fundamental processes as well as the generation of photon beams at wavelengths spanning large portions of the electromagnetic spectrum”. Toward such ends, said Chattopadhyay, “Jefferson Lab is advancing the ERL field at the fastest pace possible and is committed to working in partnership with the international community to promote the development of ERLs further as the next-generation instrument of science wherever it is feasible”. He added that “the successful emergence of the Cornell and Daresbury facilities, both collaborators with Jefferson Lab, signals a bright future ahead”.
Further reading
L Merminga, D R Douglas, G A Krafft 2003 Annual Review of Nuclear and Particle Science 53 387.
M Sherwin 2002 Nature 420 131.
M Tigner 1965 Nuovo Cimento 37 1228.
For presentations made at ERL2005 see http://conferences.jlab.org/ERL/.