In continuing CERN’s long tradition in investigating and unravelling the spin structure of the nucleon, the COMPASS experiment is also pioneering new detection techniques.
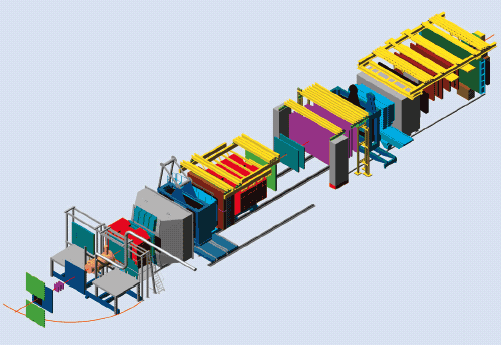
The concept for the COmmon Muon and Proton Apparatus for Structure and Spectroscopy (COMPASS) experiment first appeared on paper in a proposal submitted to CERN in 1996. A decade later, COMPASS has reached maturity, and is again taking data after the shut-down of most of the CERN accelerator complex during 2005. The year-long break provided the opportunity to carry out important upgrades to the experiment’s spectrometer, and the configuration is now very close to the one first envisaged 10 years ago. The first years of running have in the meantime already shed important light on our understanding of spin in the proton and neutron.
The goal of the COMPASS experiment is to investigate hadron structure and spectroscopy, both of which are manifestations of non-perturbative quantum chromodynamics (QCD). At large scales, QCD appears as a simple and elegant theory. However when it comes to hadrons, it is difficult to link some of their fundamental properties to quarks and gluons. Questions such as “How is the proton spin carried by its constituents?” and “Do exotics, non-qqbar mesons or non-qqq baryons exist?” still do not have clear answers. In this article we will focus on the contribution of COMPASS to the problem of nucleon spin, as it follows in the footsteps of earlier experiments at CERN.
Investigations of the spin structure of the nucleon are best performed by measuring double spin asymmetries in the deep inelastic scattering (DIS) of polarized leptons (electrons or muons) on polarized proton and neutron targets. These measurements allow the spin-dependent structure function g1(x) for the proton and for the neutron to be extracted.
The first measurements of polarized electron-proton scattering were performed at SLAC in the 1980s by the E80 and E130 Collaborations, and yielded results that were consistent with the Ellis-Jaffe sum rule. The comparison with the Bjorken sum rule is particularly important, but could not be performed at the time as the SLAC experiments did not measure the neutron. Derived as early as 1966 using current algebra tools, this sum rule relates the difference of the first moments of g1 for the proton and the neutron to GA/GV, that is, to fundamental constants of the weak interaction.
A breakthrough occurred when the European Muon Collaboration (EMC) at CERN extended these measurements to a much larger kinematic range. Using a polarized muon beam with an energy 10 times higher than at SLAC, and the largest solid polarized target ever built (about 2 l), in 1988 the collaboration reported a significant violation of the Ellis-Jaffe sum rule for the proton. In the context of the quark-parton model this implied that the total contribution of the quark spins to the proton spin is small – a major surprise that soon came to be known as the “spin crisis”. Soon after, the Spin Muon Collaboration (SMC) experiment was proposed to CERN, with the aim of improving the measurement of g1 for the proton and performing the same measurement with a polarized deuteron target.
Early results
SMC soon achieved a major accomplishment with the first measurement of g1 for the deuteron in 1992. The result, when combined with the EMC result, was in agreement with the Bjorken sum rule, and implied that the Ellis-Jaffe sum rule was also violated for the neutron. This result was particularly important because the first evidence from a competing experiment at SLAC (E142) was quite different, which suggested that either the EMC result or the Bjorken sum rule was wrong. Given the extremely sound theoretical foundations of the Bjorken sum rule, the obvious inference was that experimental finding at CERN was wrong.
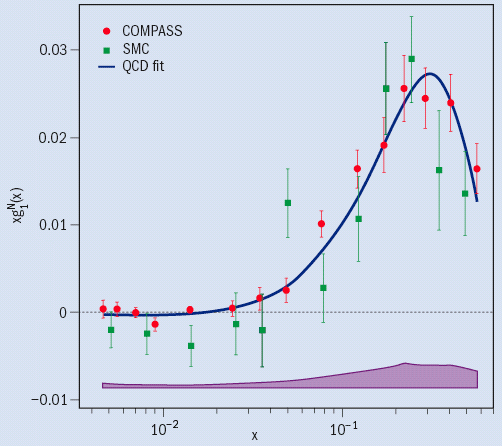
However, this was not the case. Both the EMC and the SMC experiments were right, and the original discrepancy with E142 turned out to be mostly driven by higher-order QCD corrections. So it was already safe to conclude in 1993 that the spin crisis was a well-established phenomenon for both the proton and the neutron, and that it occurred within the boundaries given by the Bjorken sum rule.
The SMC experiment also provided another important result, determining for the first time the separate contributions of the valence and sea quarks to the nucleon spin via semi-inclusive DIS measurements. Given the large range in x covered by the measurement, the polarized quark distributions could be integrated to obtain the first moments, Δq = ∫10xΔ q(x)dx, with resulting values Δuv = 0.77±0.10±0.08, Δdv = -0.52±0.14±0.09 and Δqbar = 0.01±0.04±0.03 (Adeva 1998). The polarization of the strange sea could not be accessed as this requires full particle identification, which the SMC spectrometer could not provide.
Several experiments at SLAC (E143, E154, E155, E155x), and more recently HERMES at HERA, have confirmed the results from SMC on the structure functions g1. The HERMES Collaboration has also recently reported results on the strange sea polarization.
All these measurements accurately determine ΔΣ, the contribution of both valence and sea quark spins to the nucleon spin, to be only 20%. However it was already clear in the mid-1990s that a better understanding of nucleon spin structure demanded separate measurements of the missing contributions, i.e. the gluon polarization ΔG/G and the orbital angular momentum of both the quarks and the gluons. In particular, several theoretical analyses suggested a large contribution ΔG as a solution to the spin crisis.

Progress required a new experimental approach, namely semi-inclusive DIS with the identification of the hadrons in the current jet, because the determination both of Δq and Δqbar and of ΔG requires a flavour-tagging procedure to identify the struck parton. A suggestion to isolate the photon-gluon fusion (PGF) process and measure ΔG directly had been put forward several years previously, and implied measuring the cross-section asymmetry of open charm in DIS. A new experiment, with full hadron identification and calorimetry, therefore seemed to be necessary.
At the same time, transversity, an interesting new physics case for semi-inclusive DIS measurements, was also developing rapidly. To specify the quark state completely at the twist-two level, it was realized that the transverse spin distribution ΔTq(x) has to be added to the momentum distribution q(x) and to the helicity distribution Δq(x). The DTq(x) distribution is difficult to measure because, owing to its chiral-odd nature, it cannot be measured in inclusive DIS processes. A possible way to access DTq(x) is via the Collins asymmetry, that is, an azimuthal asymmetry of the final hadron with respect to the direction of the transversely polarized quark.
Today transversity is a big issue and is a major part of the programme of many experiments. Originally the idea was much debated in the US, where it was largely responsible for the Spin Project at the Relativistic Heavy Ion Collider (RHIC) at Brookhaven. In Europe, a few enthusiasts met at a workshop in March 1993, organized by the late Roger Hess of the University of Geneva, and set down the case for a proposal (called HELP). This was submitted to CERN in the autumn, but was not accepted. However, the physics case was not given up, and, together with the measurement of ΔG, it became one of the important goals laid down in the proposal for a new experiment, COMPASS.
The COMPASS two-stage spectrometer, with particle identification and calorimetry, and the capability to handle a muon beam rate of 108 s-1, was proposed for hall 888 at CERN, after completion of the SMC experiment. Submitted in March 1996, the proposal was fully approved in October 1998 with the first physics run in 2002.
The COMPASS spectrometer
The ambitious goals of the COMPASS experiment required an entirely new spectrometer, making use of state-of-the-art detector technology and data-acquisition systems. A huge step forward had to be made in statistical accuracy and particle identification. In comparison with the SMC experiment, the incident muon flux was increased by a factor of five and a deuteron target material (6LiD) with a dilution factor roughly two times better was chosen, together accounting for a 20 fold improvement with respect to SMC. The angular acceptance for particles produced at the primary vertex was increased from ±70 mrad to ±180 mrad by a new superconducting target magnet system. The 3 m long COMPASS solenoid, with a 60 cm diameter, provides a magnetic field with a homogeneity of ±3 × 10-5 over the 1.3 m long target volume; it is being used for the first time in the 2006 run. Oppositely polarized target sections permit a direct measurement of the cross-section asymmetry.
The large acceptance requires a two-stage spectrometer. Figure 1 shows an artistic view of the apparatus, which including the beam detection fills the 100 m long experimental hall. Particles with large angles and relatively low momentum are detected in the first stage, while the fast, more central particles are analysed in the second stage, which comprises a spectrometer magnet with a stronger field and a smaller gap.
A ring imaging Cherenkov (RICH) detector provides charged particle identification. This has 116 UV-reflective mirrors forming upper and lower spherical surfaces, which focus the photons onto the upper and lower photon detectors. These detectors are multiwire proportional chambers (MWPCs) with CsI photocathodes and 80,000 pixelized read-out channels. With an area of 5.3 m2, they represent the largest such system ever deployed. A new dead-time-less APV-based read-out system for the MWPCs will be in operation for the 2006 run, while the central quarter of the system has been replaced by multi-anode photomultipliers, each with an individual lens system. This technique, which has developed enormously since the RICH was originally designed, will considerably improve background rejection and rate capability.

Photons, and therefore also neutral pions, are analysed in two electromagnetic lead-glass calorimeters, one in each spectrometer stage. The larger one, ECAL1, is being used for the first time in the 2006 run. Two hadron calorimeters reinforce particle identification and support the formation of the trigger, which is based on an array of scintillator hodoscopes and is formed in the 500 ns following the interaction. For the detector control the supervisory control and data-acquisition system selected for the LHC was chosen. Here as in many other areas COMPASS has done pioneering work that will benefit the LHC.
The high particle rates in COMPASS present a real challenge for the central particle tracking, as conventional tracking detectors would suffer from big inefficiencies. COMPASS has therefore turned to novel technologies, using micromesh gaseous structure (Micromegas) and gaseous electron-multiplier (GEM) techniques in large sizes and in large quantities for the first time. Both techniques are based on the concept of minimizing the distance that positive ions can travel by confining the gas amplification region to 50-100 μm. The Micromegas technology is based on an idea by Nobel Prize winner Georges Charpak, while the GEM is a development by Fabio Sauli’s group at CERN. Both detector types have been operating for three years in the intense muon beam of COMPASS without any sign of deterioration. Another new concept, to be employed when COMPASS uses a hadron beam, concerns cold silicon-strip detectors, which reduce the aging effect to a large extent.
To complete the overall detector assembly, precise timing information is provided by scintillating fibre trackers placed throughout the spectrometer close to the beam region. In the more peripheral region multiwire proportional chambers, drift chambers, drift tubes and straw chambers perform the large-angle particle tracking.
To cope with the high data rate arising from 250,000 read-out channels at a trigger rate of up to 20 kHz, the data acquisition has also had to enter new territory. Once the trigger is formed, the data are taken from the memory of custom-made front-end electronics, transferred to the event-building computers and stored on tape, at a rate of about 5 TB/day. Data storing and handling represent a challenge in themselves. The offline system is dealing with a raw data size of 400 TB/year, and once again COMPASS has been the guinea pig for the future experiments at the LHC. In the first three years of operation 20 billion events have been put on tape and processed several times.
The first important results have already been obtained from the huge amount of data collected by COMPASS. The g1 structure function of the deuteron has been measured with unprecedented accuracy in the low-x region, improving by at least a factor of six the precision of the SMC measurement (Ageev et al. 2005). Essential data for g1 come from SLAC and HERA (and recently from Jefferson Lab), but the CERN experiments are unique at low x, giving an invaluable contribution to the evaluation of the first moment of g1 and thus ΔΣ, which requires the data to be extrapolated to x = 0. The Q2 evolution of g1 also contains important information on ΔG. Here the COMPASS data have a particular impact, since they lie at the high-Q2 end of the available experimental information. The new preliminary COMPASS data are shown in figure 2, together with the SMC data and the result of a recent QCD fit to the world data set comprising 230 data points. Recent fits now suggest rather small values for ΔG.
Competition breeds innovation
Direct measurements of ΔG are particularly important. In this field COMPASS is in competition with the experiments at RHIC, which look at the cross-section asymmetry of prompt photons or π0s produced in collisions between polarized protons to estimate ΔG. Three independent measurements have been performed by COMPASS using the cross-section asymmetry of (i) open-charm production (detecting either D or D* charmed mesons); (ii) high-pT hadron pairs in DIS events (Q2 > 1 GeV2); and (iii) high-pT hadron pairs in photoproduction (Q2 < 1 GeV2). In all these processes, the PGF contribution is important, but the background is different. COMPASS is unique in the open-charm measurement. The high-pT hadron-pairs method was invented within the COMPASS Collaboration while setting up the experiment, and has already been applied to estimate ΔG by HERMES (all Q2) and SMC (Q2 > 1 GeV2).
The COMPASS results (Ageev et al. 2006) are shown in figure 3 together with the results from the other collaborations and next-to-leading order QCD fits corresponding to a first moment of ΔG at Q2 = 3 GeV2 of 2.5, 0.6 and 0.2 for the maximum, standard and minimum scenarios, respectively. Small values for ΔG are favoured, and method (iii) from COMPASS now provides fairly precise information.
Another prime objective of COMPASS is the investigation of transverse spin effects
Another prime objective of COMPASS is the investigation of transverse spin effects. The transversity distributions are difficult to measure because they can be obtained from the transverse spin asymmetries only after unfolding the Collins effect. This requires a global analysis of transverse spin asymmetries of several identified hadrons produced in semi-inclusive DIS, as well as the analysis of spin asymmetries in e+e– → 2 hadrons, as currently measured by the BELLE Collaboration. In this worldwide effort, COMPASS has provided the first asymmetry data for the deuteron. The measured asymmetries are very small (Alexakhin et al. 2005) (figure 4). Taking into account the fact that the HERMES Collaboration has measured non-zero Collins asymmetries on a transversely polarized proton target, the COMPASS result very likely points to a cancellation between proton and neutron, much as for the longitudinal case where g1 for the neutron has the opposite sign to g1 for the proton. Further investigations of transverse-spin effects are related to ongoing measurements of the Sivers asymmetry, the two-hadron interference function and the Λ polarization transfer.
The search continues
The COMPASS analysis group is currently investigating many more physics channels. The wealth of data allows for the search for new states in quasi-real photoproduction; the recently announced pentaquark states Θ+(1530) and the Ξ–(1860) have been looked for here, but so far with negative results. Very large samples of Λ hyperons allow the study of reaction mechanisms and polarization transfer. In a similar way, the measurement of the spin density matrix of vector mesons (ϕ, ρ, ρ’) provides stringent tests on reaction mechanisms, such as s-channel helicity conservation; a phase-shift analysis of the π+ π+ π– π– has recently begun.
Running-in the new spectrometer has taken some time. Owing to the non-availability of the COMPASS polarized-target magnet, the experiment has until now used the SMC target system, which has a much smaller acceptance. Physics data were collected in 2002, 2003 and 2004, doubling the amount of data each year, thanks to several improvements in the apparatus. This should again be the case for the 2006 run, when the new COMPASS polarized-target magnet is used for the first time.
COMPASS is scheduled to take data until the end of the decade both for its hadron programme and for its muon programme with a polarized proton target. An Expression of Interest has been put forward for a new experimental programme, based on an upgraded COMPASS spectrometer (COMPASS-II) and an even higher beam flux. The emphasis of the future programme will be on the still unknown orbital angular momentum of the partons inside the nucleon. This will be addressed in two different ways, first by the measurement of generalized parton distributions in deeply virtual Compton scattering and in hard exclusive meson production processes, and second by a precise determination of the first moments of the transversity distributions that are linked to the orbital angular momentum via the Bakker-Leader-Trueman sum rule. Of course, an important part of the COMPASS-II programme will still be spectroscopy, where many open questions remain.