Measurements of the properties of the Higgs boson discovered in 2012.

Since the inception of the LHC, a central part of its physics programme has been aimed at establishing or ruling out the existence of the Higgs boson, the stubbornly missing building block of the Standard Model of elementary particles. After the discovery of a Higgs boson by the ATLAS and CMS experiments was announced in July 2012, the study of its properties became of paramount importance in understanding the nature of this boson and the structure of the scalar sector. Given the measured mass of the Higgs boson, all of its properties are predicted by the theory, so deviations from the predictions of the Standard Model could open a portal to new physics.
The CMS collaboration recently completed the full LHC Run 1 data analysis in each of the most important channels for the decay and production of the Higgs. Bosonic decays such as H → ZZ → 4 leptons (4l), H → γγ, and H → WW → lνlν, and fermionic decays such as H → bb, H → ττ and H → μμ, were studied, and the results have been published. All of the analyses are based on the proton–proton collision data collected in 2011 and 2012 at the LHC, corresponding to 5 fb–1 at 7 TeV and 20 fb–1 at 8 TeV centre-of-mass energy. The di-boson channels are observed with significance close to or above 5σ. The Standard Model’s hypothesis of 0+ for the spin-parity of the observed Higgs boson is found to be favoured strongly against other spin hypotheses (0– ,1±, 2±). The comparison of off-shell and on-shell production of the Higgs boson in the ZZ channel also sets a constraint on the natural width of the Higgs boson that is comparable to the width expected in the Standard Model. Furthermore, evidence is established for the direct coupling to fermions, with significance above 3σ for the decay to ττ.
The first preliminary results on the full Run 1 data were presented by CMS last July at the International Conference on High Energy Physics
The combination of all of the production and decay channels provides the opportunity to obtain a global view of the most important Higgs-boson parameters, and to disentangle the contributions to the measured rates from the various processes. The first preliminary results on the full Run 1 data were presented by CMS last July at the International Conference on High Energy Physics in Valencia. Now, the collaboration has submitted the final “Run 1 legacy” results on the Higgs boson for publication. The results combining individual channels are remarkably coherent.
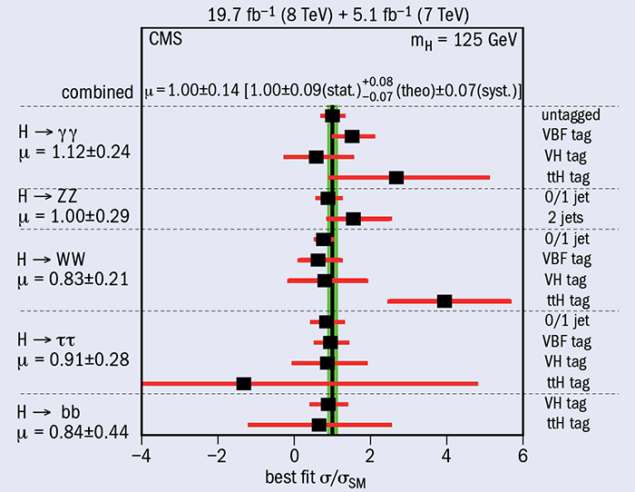
A first major outcome of the combination is a precise measurement of the mass of the Higgs boson. This is achieved by exploiting the two channels with the highest resolution: H → γγ and H → ZZ → 4l. Thanks to the high precision and accurate calibration of the CMS electromagnetic calorimeter, the H → γγ channel gives a most precise single-channel measurement of MH = 124.70±0.34 GeV. Using the combination with the H → ZZ → 4l channel, the final measurement of MH = 125.03+0.29–0.31 GeV is obtained with an excellent precision of two per mille. The measurements in the two channels (figure 1) are compatible at the level of 1.6σ, indicating full consistency with the hypothesis of a single particle. The measured value of the mass is used for further studies of the Higgs-boson’s couplings. It is worth noting that the uncertainty is still dominated by the statistical uncertainty and will therefore improve in Run 2.
The various measurements performed at the two centre-of-mass energies are carried out in a large number (around 200) of mutually exclusive event categories. Each category addresses one or more of the different production and decay channels. Four production mechanisms are considered. Gluon–gluon fusion (ggH) is a purely quantum process, where a single Higgs boson is produced via a virtual top-quark loop. In vector-boson fusion (VBF), the Higgs boson is produced in association with two quarks. Lastly, in VH- and ttH-associated production, the Higgs boson is produced either in association with a W/Z boson or with a top–antitop quark pair. The main decay channels are indicated on the left of figure 2, which shows the measurement of the signal strength μ, defined as the ratio of the measured yield relative to the Standard Model prediction. All of the measurements are found to be consistent with μ = 1, which by definition indicates consistency with the prediction. The combination of all of the measurements gives an overall signal strength of 1.00±0.13. The figure also shows the signal strengths measured for the different decay tags. All of the combinations are obtained using simultaneous likelihood fits of all channels, with all of the systematic and theory uncertainties profiled in the fits.
Signal strengths compatible with Standard Model expectations are also found for each of the production mechanisms, with an observation of ggH production at more than 5σ and evidence for VBF, VH and ttH production at close to or above 3σ.
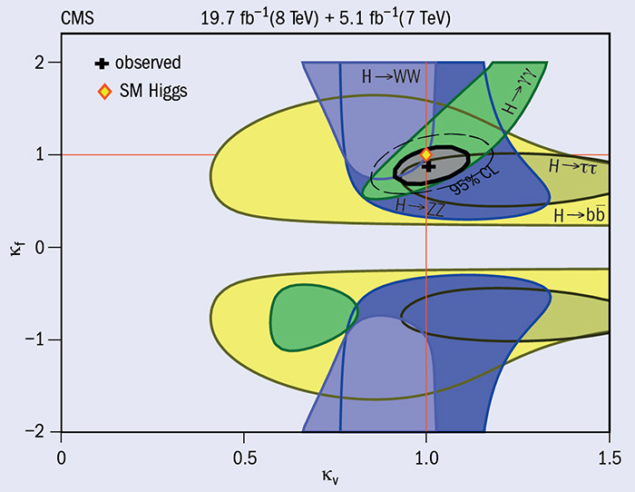
Another set of tests of consistency with the Standard Model consist of introducing coupling modifiers, κ, that scale the Standard Model couplings. The simplest case is to allow one scaling factor for the coupling of the Higgs boson to the vector bosons (κV) and one for the coupling to fermions (κf), and to resolve the loops – namely gluon–gluon fusion and γγ decay – using Standard Model contributions only.
Figure 3 shows the 1σ contours obtained from the different decay channels in the plane κf versus κV, and from their combination. The only channel that can distinguish between the different relative signs of the two couplings is H → γγ, because of the negative interference between the top-quark and W-boson contributions in the loop. The combination (thick curve) shows that the measurement is consistent within 1σ with κV = κf = 1, while the opposite sign hypothesis, κV = –κf = 1, is excluded with a confidence limit (CL) larger than 95%.
Many other tests of modified couplings with respect to the Standard Model have been carried out, and all of the results indicate consistency with the predictions. For instance, the so-called “custodial” symmetry that fixes the relative couplings κW/κZ of the Higgs boson to W and Z bosons is verified at the 15% precision level and the couplings to fermions of the third family are verified at the 20–30% precision level.

Fig. 4. Graphical representation of the results obtained from likelihood scans for a model where the gluon and photon loop-interactions with the Higgs boson are resolved in terms of other Standard Model particles. The dashed line corresponds to the Standard Model expectation. The inner bars represent the 68% CL intervals, while the outer bars represent the 95% CL intervals. The ordinate differs between fermions and vector bosons to take account of the expected Standard Model scaling of the coupling with mass, depending on the type of particle. The continuous line shows the result of the coupling–mass fit, while the inner and outer bands represent the 68% and 95% CL regions.
The Higgs boson is tightly connected with the mechanism for generating mass in the Standard Model: the Yukawa couplings for the fermions are predicted to be proportional to the mass of the fermions themselves, while the gauge couplings to the vector bosons are proportional to the masses squared of the vector bosons. Figure 4 illustrates this by showing the couplings to the Standard Model particles as a function of the mass of their masses. All of the measurements are in excellent agreement with the expected behaviour of the couplings, indicated by the black line. In this plot the H → μμ channel is also included and, even though it currently has a large uncertainty, it is consistent with the fitted line. This demonstrates beautifully that the Higgs boson is linked to the fundamental field at the origin of the masses of particles.
Summary and conclusions
CMS has just submitted for publication the final Run 1 measurements of the properties of the Higgs boson – mass, couplings and spin-parity parameters – with the highest precision allowed by the current statistics. So far, all of the results are found to be consistent, within uncertainties, with the newly established scalar sector, just as predicted for the spontaneous electroweak symmetry breaking in the Standard Model. The measurements provide overwhelming evidence that the observed Higgs-boson couples to other particles in a way that is consistent with the Standard Model predictions. After achieving the major milestone of completing all of the most important Run 1 Higgs-boson measurements, the CMS experiment will now direct its efforts towards the exploitation of the upcoming LHC run (Run 2) at a centre-of-mass energy of 13 TeV. The new energy frontier promises increased reach into the Higgs sector, but also a unique look at a totally new, unchartered territory.