Conventional dogma says that the Big Bang was the beginning of everything. Here, Gabriele Veneziano of CERN challenges this view. He believes that the Big Bang is the biggest thing that the universe has seen, but that it did not take place at time zero.
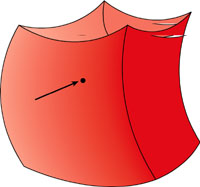
The idea that the universe, and time itself, originated from a primordial explosion – the Big Bang – has transcended the boundaries of scientific knowledge to become a key part of modern culture. But is this belief completely justified? An alternative explanation, suggested by recent developments in theoretical physics, holds that the Big Bang was just one of many transitions in the history of the universe, though possibly the most dramatic. In this new scenario, a long prehistory slowly prepared the Big Bang in much the same way that long periods of steady evolution precede the collapse or explosion of stars. But what is wrong with the traditional dogma?
Singularity
Standard cosmology, based on Einstein’s Theory of General Relativity, states that some 10 billion years ago the universe was just a mathematical point. As such, not only did it have exactly zero size, but physical quantities, such as temperature, energy density, pressure and the resulting curvature of space-time were all infinite. Indeed, the Big Bang is the most dramatic example of a phenomenon that is often encountered in field theories like General Relativity, when the predictive powers of the theory break down. The phenomenon is known as a singularity. Asking what happened before that singular instant, which is conventionally defined as time zero, doesn’t make any sense. There was no “before”. At least, that’s what we are told.
When hard pressed, cosmologists admit that extrapolating the equations of General Relativity back to time zero is not justified. Even the most optimistic will agree that what happened around and before 10-43 seconds was greatly affected by quantum effects that General Relativity happily ignores. (10-43 seconds is the so-called Planck time: the timescale first constructed by Max Planck out of the speed of light, Planck’s constant and Newton’s constant of gravitation. At accelerator energies, the gravitational force between two elementary particles is much weaker than the other forces of physics. However, at the Planck scale the effects become comparable.)
Thus, to be on the safe side, we should ask how the universe would have looked not at t = 0 but, say, at t = 3 x 10-43 seconds, possibly a bit later. The answer turns out to be quite embarrassing. According to General Relativity, the many-billion-light-year universe that we observe today was then only one millimetre across. This size, though tiny compared with the present dimensions, was huge compared with the other relevant distance at the time: the distance travelled so far by light: 10-32 centimetres.
Cosmological puzzles
The enormous ratio of these two scales, 1031, is both a mystery and the source of many unsolved cosmological puzzles (the so called homogeneity, entropy and flatness problems). These problems have been nicely summarized by Roger Penrose in an illustration in his compelling book The Emperor’s New Mind, in which God selects with unimaginable care the initial state of the universe. In particular, this initial state had to contain a huge amount of matter/energy distributed in a very orderly fashion. This was as improbable as finding, at one moment, all of the molecules of the Earth’s atmosphere above the North Pole, and nowhere else.

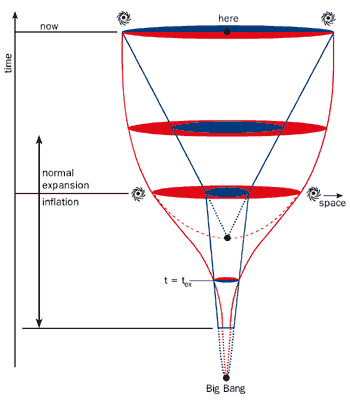
Whenever similarly odd situations are encountered in physics, the obvious question to ask is: “Can we understand them as the result of an evolution from something more ‘natural’?” However, if we assume that the Big Bang was the beginning of everything, there is simply no “past” available to us for assuming those more natural initial conditions. The standard solution to these cosmological puzzles is to invoke the hypothesis that something new a relatively long period of extremely rapid expansion of the universe, inflation took place after the Big Bang and cured its deficiencies. Unfortunately, even after some 18 years of hard work, inflation still lacks a deep theoretical understanding and contains a large degree of arbitrariness, and is therefore difficult to test.
Alternatively, one should appeal to processes that happened around or at the Planck time, but then, as was mentioned earlier, one has to include sizable quantum effects. Indeed, it looks quite plausible that the singularity problem and the puzzles of standard cosmology should somehow be related, and should be simultaneously solved by quantum effects. However, until recently, there has been no way of dealing with these kinds of question. There was no scheme combining General Relativity and quantum mechanics into a consistent theory of quantum gravity.
This situation changed some 15 years ago with the advent of superstring theory, which was itself a re-elaboration of a model developed at CERN about 30 years ago. The way in which strings cope with the problems met by the conventional approach is subtle, but not difficult to describe. In string theory, conventional point-like particles are replaced by small, one-dimensional objects called strings. The typical size of a string, which is dictated by quantum mechanics, defines, through the speed of light, a characteristic timescale that turns out to be about an order of magnitude larger than the Planck time: ts = 10-42 seconds.