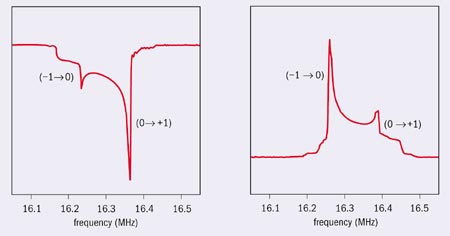
Polarized solid targets have been used in nuclear and particle-physics experiments since the early 1960s, and with the development of superconducting magnets and 3He/4He dilution refrigerators in the early 1970s, proton-polarization values of 80-100% have been routinely achieved in various target materials at two standard magnetic field and temperature conditions (2.5 T; < 0.3 K and 5 T; 1 K). Due to the much lower magnetic moment of the deuteron compared with that of the proton, deuteron polarization values have been considerably lower, typically 30-50%. Now, however, research at the University of Bochum is yielding materials with deuteron polarizations as high as 80%.
During the past 10 years, polarized solid targets have been successfully used at CERN and SLAC to investigate the spin structure of the nucleon. For this purpose hydrogen- and deuterium-rich compounds such as butanol, deuterated butanol (D-butanol), ammonia (NH3 and ND3) and lithium deuteride (6LiD) have served as proton and neutron target materials, respectively. The basic technique used to obtain a high polarization of the nuclear spins in the solid targets is to transfer the almost complete polarization of electrons at high magnetic fields and low temperatures to the nuclei via a microwave field with a frequency close to the electron Larmor frequency. This process – called dynamic nuclear polarization (DNP) – works for any nucleus with spin. Because all the target materials used are diamagnetic compounds, some amount of paramagnetic impurities (radicals or crystalline defects with unpaired electron spins) have to be implanted into the host material (doping). However, the efficiency of DNP in achieving high polarizations depends strongly on the nature of the paramagnetic centre and on the interactions to which the respective unpaired electron is exposed.
According to spin temperature theory, a narrow electron paramagnetic resonance (EPR) line enables the creation of high inverse spin temperatures – and thus of high nuclear polarizations. Guided by this prediction, a team at the University of Bochum has therefore been performing a systematic study of target-material doping for several years, using EPR spectroscopy to study the characteristics of the different paramagnetic dopants.
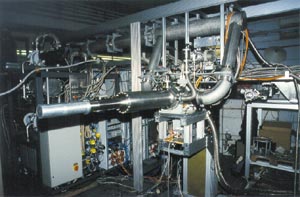
In the search for radicals with a small EPR line width, any effects that tend to broaden the line have to be minimized. The anisotropic g-factor of the unpaired electron is a particular danger, because its influence on the line width increases with increasing magnetic field. Hyperfine interactions of the electron also cause broadening. Radiation doping of deuterated materials therefore appears useful because the resulting paramagnetic centres combine a small g-factor anisotropy with weak deuteron hyperfine splitting.
A good example of the useful paramagnetic centres discovered so far is the so-called F-centre in 6LiD, in which the EPR line width is almost entirely given by the magnetic interaction of the F-centre electron, with its six neighbouring 6Li nuclei. A maximum deuteron polarization of 56% at 2.5 T is already being achieved with the target for the COMPASS experiment (NA58) at CERN, which was developed and produced at Bochum. Measurements at Saclay are also showing that even higher polarization values can be obtained in this material at higher magnetic fields.
The research at Bochum has also concentrated on the improvement of deuterated alcohol targets. These play an important role in experiments at intermediate beam energies because all the nuclei in these materials (apart from deuterium) are spinless – unlike 6Li which has spin 1. The first breakthrough in this field came with the application of the radiation doping method to D-butanol. In this way, paramagnetic defects with characteristics very similar to those of the F-centres in 6LiD could be created. Although a systematic investigation concerning the optimum irradiation dose has not yet been performed for this material, deuteron polarizations of 54% at 2.5 T and 71% at 5 T have already been reached.
A further substantial increase of the deuteron polarization has been achieved in both D-butanol and D-propanediol, chemically doped with radicals of the trityl family (which was developed and delivered by Amersham Health, Malmö). Basically, the paramagnetic part of these molecules consists of a methyl-type radical with the three H-atoms replaced by larger but spinless complexes. They possess a very small EPR line width compared with those of the commonly used nitroxide radicals, porphyrexide and TEMPO. Both D-butanol and D-propanediol doped with radicals of the trityl family could be polarized up to around 80% at a magnetic field of 2.5 T. The nuclear magnetic resonance (NMR) signals for positively polarized butanol-d10 and negatively polarized 1,2-propanediol-d8 are shown in figure 1. They consist of two lines corresponding to the two transitions (m = +1→m = 0) and (m = 0→m = -1) of a spin-1 system, respectively. The resonances are separated by the so-called quadrupole splitting, which is a consequence of the interaction of the deuteron quadrupole moment with the lattice electrical field gradient at the site of the deuteron. The measurement of the intensity ratio of the two transitions provides a very accurate method for determining the polarization.
These new developments will allow polarization experiments to be performed on the deuteron and neutron with a much higher precision than was previously possible. Doubling the maximum polarization means doubling the statistical accuracy for the same measuring time. Alternatively, for a given accuracy, the measuring time required is reduced by a factor of four. For these reasons, trityl-doped D-butanol was recently successfully used for the neutron part of an experiment to study the Gerasimov-Drell-Hearn sum rule at the Mainz microtron, MAMI, using a polarized tagged photon beam. Figure 2 shows the horizontal dilution refrigerator developed by the Bonn Polarized Target Group for this particular experiment. For the first time, after more than 40 years of polarized solid targets, it is now possible to perform these kinds of experiments with low-intensity beams to the same precision and over the same time span, no matter whether the proton or the neutron is the subject of investigation.