A look at some unexpected results from proton–lead collisions.
Résumé
ALICE : la matière nucléaire froide est-elle vraiment froide ?
En septembre 2012, pour la première fois, des collisions proton-plomb ont eu lieu au LHC ; une exploitation proton-plomb de plus grande durée et de plus grande intensité a eu lieu en janvier-février 2013. L’expérience ALICE a permis de recueillir des données au cours des deux périodes d’exploitation. Outre les résultats attendus, de nombreuses observations inattendues ont été faites. Les surprises proviennent de similitudes de plusieurs observables entre collisions proton-plomb et collisions plomb-plomb au LHC. Ces similitudes pourraient indiquer l’existence de phénomènes collectifs dans les collisions proton-plomb avec forte multiplicité, et, finalement, la formation de l’état déconfiné de la matière connu sous le nom de plasma quark-gluon.

In September 2012, the LHC provided proton–lead (pPb) collisions for the first time, two years after its heavy-ion collisions opened a new chapter in exploration of the properties of the deconfined, chirally symmetrical state of matter known as quark–gluon plasma, or QGP (CERN Courier October 2013 p17). Until then, measurements in lead–lead (PbPb) collisions had been typically compared with the corresponding proton–proton (pp) results to assess the properties of QGP. The strategy proved to be successful but remained incomplete. Indeed, within such an approach, the initial wave function of the colliding nuclei is not taken into account. This consideration was the primary motivation for including the measurements in pPb collisions as part of the heavy-ion programme at the LHC, with the expectation of being able to disentangle effects arising from the structure of the initial state of the collision – often dubbed “cold nuclear matter” effects – from the final-state effects related to the medium created, presumably, only in PbPb collisions.
In addition, understanding the structure of the initial state is interesting in its own right, because at the LHC energy, experiments probe the structure of the nucleus in a novel and unexplored QCD regime of very low values of the longitudinal parton-momentum fraction (x <10–3). In this kinematic region, the extremely high gluon density is expected to saturate by means of strongly non-linear coherent processes, leading theorists to predict the existence of yet another pre-collision state of matter – the so-called colour glass condensate (CGC).
ALICE collected data from pPb collisions at the LHC during both the short pilot run in September 2012 (CERN Courier November 2012 p6) and the longer high-luminosity run in January and February 2013 (CERN Courier March 2013 p5). The two-in-one design of the LHC magnets imposes the same magnetic rigidity for the two beams, necessitating an asymmetrical set-up in beam energy for pPb collisions. So in these runs, the energy in the nucleon–nucleon centre-of-mass system was √sNN = 5.02 TeV, with the centre of mass shifted in the direction of the proton beam by about half a unit in rapidity with respect to the laboratory system.
The results from the basic control measurements, the charged-particle multiplicity density and transverse-momentum spectrum, were in agreement with expectations from theoretical models that incorporate present knowledge of the function describing the longitudinal distribution of partons inside the nucleus. In addition, when compared with the results obtained for pp collisions at 2.76 and 7 TeV, on one hand the charged-particle multiplicity in pPb collisions was found to scale roughly with the mean number of nucleons participating in the collision, as expected for particle production through soft processes. On the other hand, the particle spectrum at large transverse momenta – beyond 3–4 GeV/c – was found to scale with the mean number of binary nucleon–nucleon collisions, as expected for particle production through hard processes (CERN Courier December 2012 p6). Extending this latter measurement to charged jets and charmed mesons (figure 1) revealed no deviation from binary nucleon–nucleon scaling outside the large experimental uncertainties. The above observations confirm that the suppression of high transverse-momenta hadrons and jets observed in central PbPb collisions can be attributed to a final-state effect, namely, the energy loss experienced by partons traversing the medium created in these collisions.
As figure 2 shows, the measured rapidity distribution of the J/ψ charmonium state in pPb collisions, when compared with that measured in pp collisions, exhibits a moderate suppression in the forward hemisphere (positive values of rapidity, in the direction of the proton beam), while there is no suppression and even a slight enhancement in the backward hemisphere (negative values of rapidity, in the direction of the lead beam). These results can be well described by models that invoke the cold-nuclear-matter effects only and seem to disfavour the CGC-based models.
The first surprise came from the study of two-particle correlations in high-multiplicity pPb events. A surprising near-side, long-range (elongated in pseudorapidity) correlation, forming a ridge-like structure observed in high-multiplicity pp collisions, was also found in high-multiplicity pPb collisions, but with a much larger amplitude (CERN Courier January/February 2013 p9). However, the biggest surprise came from the observation that this near-side ridge is accompanied by an essentially symmetrical away-side ridge, opposite in azimuth (CERN Courier March 2013 p6). This double ridge was revealed after the short-range correlations arising from jet fragmentation and resonance decays were suppressed by subtracting the correlation distribution measured for low-multiplicity events from the one for high-multiplicity events.

Similar long-range structures in heavy-ion collisions have been attributed to the collective flow of particles emitted from a thermalized system undergoing a collective hydrodynamic expansion. Early interactions produce pressure gradients that translate the spatial anisotropy in the overlapping region of the nuclei into an anisotropy in momentum space. This anisotropy can be characterized by means of the vn (n = 2, 3, …) coefficients of a Fourier decomposition of the single-particle azimuthal distribution.
Recently, the analysis has been extended to four-particle correlations, which have been proved in heavy-ion collisions to be much less sensitive to jet-related correlations. Again, the similarity with results obtained in PbPb collisions is striking: the v2 harmonic coefficients measured in pPb are similar to the ones obtained in PbPb collisions at comparable event multiplicities, as is their transverse momentum dependence – a continuous increase up to a transverse momentum of 2–3 GeV/c, followed by a gradual decrease owing to the increasing contribution from the jet fragmentation. The latter effect arises because the harmonic coefficients measure the strength of the particle correlations with respect to a symmetry plane, while the jet fragments are expected to be only slightly correlated via energy losses to that plane, because they are not expected to participate in the collective motion of the system.
To test the possible presence of collective phenomena further, the ALICE collaboration has extended the two-particle correlation analysis to identified particles, checking for a potential mass ordering of the v2 harmonic coefficients. Such an ordering in mass was observed in heavy-ion collisions, where it was interpreted to arise from a common radial boost – the so-called radial flow – coupled to the anisotropy in momentum space. Continuing the surprises, a clear particle-mass ordering, similar to the one observed in mid-central PbPb collisions (CERN Courier September 2013 p6), has been measured in high-multiplicity pPb collisions.
These similarities are not the only ones. By measuring the dependence on the event multiplicity of the identified-particle transverse-momentum spectra or the averaged transverse momentum (CERN Courier September 2013 p10), a significant hardening of the spectra has been observed with increasing multiplicity. Moreover, the hardening is found to be stronger for heavier particles. Such an observation is interpreted in PbPb collisions as a signature of radial flow. The blast-wave fit, with parameters associated with the kinetic freeze-out temperature and the mean transverse-expansion velocity, describes the shape of the particle spectra, as well as the v2 coefficient (figure 3).
The value and the multiplicity dependence of these two parameters are similar to the ones measured in PbPb collisions. Although models that consider a hydrodynamic evolution of the colliding system can describe this feature satisfactorily, an alternative explanation has been put forward: colour reconnection. This mechanism, implemented in the PYTHIA event generator, can be seen as a final-state interaction between outgoing partons originating from different hard-scattering processes and might result in effects that are qualitatively similar to particle-flow correlations.
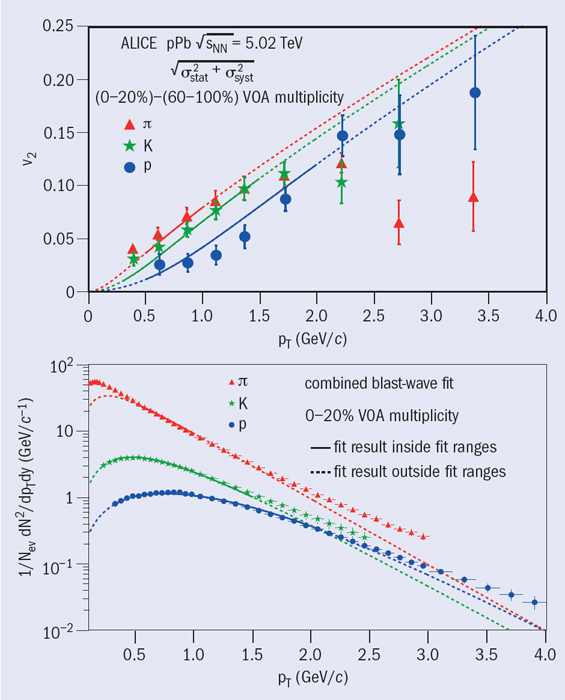
The final surprise, so far, comes from the charmonium states. Whereas J/ψ production does not reveal any unexpected behaviour, the production of the heavier and less-bound (2S) state indicates a strong suppression (0.5–0.7) with respect to J/ψ, when compared with pp collisions. Is this a hint of effects of the medium? Indeed, in heavy-ion collisions, such a suppression has been interpreted as a sequential melting of quarkonia states, depending on their binding energy and the temperature of the QGP created in these collisions.
Taking all of these observations together and in view of the astonishing similarities between pPb and PbPb collisions, it is tempting to raise the obvious question: is QGP formed in high-multiplicity pPb collisions? By considering the measured particle multiplicity and the small size of the colliding system, it can be deduced that the initial energy density in high-multiplicity pPb events exceeds the one measured in PbPb collisions, and therefore the critical value for the QGP phase transition. However, could such a small and short-lived system reach thermal equilibrium fast enough to form a QGP-like droplet?
Part of the answer comes from the measured properties of QGP. As the estimated ratio of shear viscosity to entropy is close to the quantum limit for a perfect liquid, it is possible to deduce a value of the mean free path of constituents inside QGP that is several times smaller than the typical pPb system size of 1–2 fm. However, other explanations of the origin of the observed collective-like phenomena cannot be excluded. Rather as the colour-reconnection mechanism can explain the observed hardening of the transverse-momentum spectra with multiplicity, other models developed within the CGC framework can describe the dependence of two-particle correlations on the event multiplicity and the identified particle transverse momentum, but they are less successful in describing results from four-particle correlations and identified-particle correlations. Of course, this does not exclude the melting of CGC during the initial stage of the collision, followed by thermalization of the system and hydrodynamic flow.
To summarize this first pPb measurement campaign, expected results were widely accompanied by unanticipated observations. Among the expected results is the confirmation that proton–nucleus collisions provide an appropriate tool to study the partonic structure of cold nuclear matter in detail. The surprises have come from the similarity of several observables between pPb and PbPb collisions, which hint at the existence of collective phenomena in pPb collisions with high particle multiplicity and, eventually, the formation of QGP.
A deeper insight into the dynamics of pPb collisions can come from exclusive measurements classifying events according to their impact parameter and extracting the physics observables in intervals of collision centrality. In PbPb collisions, the centrality estimators typically make use of the particle multiplicity or total transverse-energy measured in various pseudorapidity intervals, and the estimator value is then transformed into a number of binary nucleon–nucleon collisions through the Glauber model, which provides a classical representation of the initial geometry of the colliding system. In pPb collisions, the event classification into centrality intervals is strongly biased, owing to fluctuations of the measured particle multiplicity for a given number of binary nucleon–nucleon collisions.
In general, central (peripheral) events have larger (smaller) particle multiplicity per participating nucleon than average, the particle-production source being parton scattering. At LHC energies, such fluctuations are large because they are introduced by multi-parton interactions whose amount fluctuates strongly among events of similar centrality. Therefore the event classification using the conventional centrality estimators breaks the scaling of the measured particle production with the number of binary nucleon–nucleon collisions. This has been demonstrated by comparing the charged-particle spectra at mid-rapidity in centrality intervals defined by various centrality estimators available in ALICE.
Nevertheless, if a proper centrality determination can be established, all of the above-mentioned physics observables will be studied in centrality intervals to answer in particular the question of whether the jet-quenching phenomenon is present in central pPb collisions. At the same time, while the LHC is being prepared for its second phase of operation, the ALICE collaboration continues to analyse the precious sample of pPb collision data already recorded.