CERN’s Proton Synchrotron achieved its first high-energy beams 40 years ago. The pioneers at CERN had dared to follow a new, untested route in a bid to become the world’s highest energy machine. Now, 40 years later, the valiant Proton Synchrotron remains the ever-resourceful hub of an unrivalled particle beam network.

On 24 November 1959, CERN’s new Proton Synchrotron accelerated protons through the dreaded “transition energy” barrier and on to achieve the nominal energy of 24 GeV. Could any of those rejoicing on that historic day have thought that their machine would still be alive and well 40 years later, or that it would be the hub of the world’s largest complex of accelerators, at the centre of European high-energy physics? If such thoughts were on their minds, they certainly would not have extended so far in time or in performance.
When operation for physics began in 1960, the Proton Synchrotron (PS) delivered 1010 protons per pulse. Since then, 40 years of relentless effort has improved the performance more than a thousandfold. Today the PS delivers 3 x 1013 protons per pulse.
The early history – the decision to build the PS, the courageous step from a weak-focusing to a strong-focusing synchrotron, its design, construction and legendary start-up – has been told before. Instead we look at the evolution that has led to today’s PS, the complex that has grown around it and the assured future that awaits it.
History 1959-1999: protons
The performance of the PS has increased, partly through patient optimization and partly owing to specific steps taken to cope with CERN’s evolving physics programme. In June 1960 the Parameter List showed a “best performance” of 6 x 1010 protons per pulse and further progress was rapid (figure 2).
Physics quickly benefited from Europe’s new high-energy proton source. In the first year of operation, internal targets supplied four experiments in the South Hall with secondary particles for more than 1000 h. More beamlines came into operation in 1961. From 1963, fast and slow extraction supplied primary protons to the East Area. Between 1972 and 1975 they were supplied to the West Area.
Although the intensity had increased a hundredfold by 1964, the many particle-hungry users, and the prospect of having to supply the new ISR proton collider, triggered an improvement programme.
A new main magnet power supply would double the repetition rate. A new Booster – four superimposed synchrotron rings, one quarter of the PS diameter, taking 50 MeV protons from the Linac up to 800 MeV – would raise the PS space-charge limit by a factor of 10, to 1013 protons. Construction of the Booster began in 1968 and was completed in 1972. With it came an increase in PS intensity, which was welcomed by the ISR and the Gargamelle bubble chamber. A further increase in intensity came in 1978, when the new Linac 2 replaced the original one. Today the Booster more than triples its design intensity, routinely delivering 3 x 1013 protons per pulse, to the delight of the ISOLDE radioactive-ion beam users.
In striving for higher intensities, several obstacles had to be overcome. One was passing the transition energy in the PS causing the dilution of longitudinal phase-space density. This was remedied in 1970 by the “Q-jump” and then the “gamma-transition jump”, in which pulsed quadrupoles make the protons traverse transition much faster. This trick came just in time for the much higher intensities generated by the Booster.
In 1976 the new SPS synchrotron became a welcome client. In 1970, in a deadlock over site selection for the new “300 GeV project”, John Adams had proposed attaching it to the existing CERN site and use the PS as the injector, thereby consolidating the future of the machine he had built and of CERN as a whole.

The variety of users and their demands generated a need for the PS to deliver different beams on successive cycles. This was called pulse-to-pulse modulation, with a number of different cycles grouped into a “supercycle” (figure 1). It was a great challenge for the long sequence of ever more powerful controls computers, the first having been a 1967 IBM 1800.
When, in December 1991, the council declared that the LHC was the “right next machine” for CERN, the PS had to prepare for yet another client – a very demanding one. The LHC would need proton beams of unprecedented density. For the Booster and the PS, this entailed a number of drastic changes, the validity of which was tested extensively at the end of 1993.
The radiofrequency systems in the Booster and PS were radically modified for acceleration on new harmonic numbers. Intricate radiofrequency gymnastics for splitting and merging bunches were also introduced (figure 3).

To obtain high transverse beam densities, the blow-up of emittances must be strictly limited. In 1999 the harmful effects of space charge in the PS were reduced by raising the Booster energy to 1.4 GeV. To preserve emittance tightly requires measuring it accurately. Existing diagnostic tools were sharpened and new ones added. Today, automatic beam steering alleviates the burden of optimization and will be an essential tool in meeting the LHC stringent emittance requirements.
Acceleration of ever higher intensities brings higher radiation doses to the machine components. The first radiation damage was recorded as early as 1964, after a total of 1.1 x 1019 protons had been accelerated. Strict measures were taken to reduce losses – figure 4 shows how successful these measures were – otherwise the total of 1.56 x 1021 protons delivered so far would never have been reached. (The rest mass of all of the protons accelerated during 40 years of operation amounts to a mere 2.6 mg.)
Protons are not the only particles supplied. In 1964 the Linac produced deuterons of 12 MeV/nucleon and the PS accelerated them for a few milliseconds. In 1976 and 1977, deuterons were accelerated and transferred to the ISR for d–d collisions. When Linac 2 came into operation for protons, Linac 1 was left free for more ion studies.

In 1980, alpha particles were provided for the ISR. Heavier ions, oxygen and sulphur, were sent to the SPS. This whetted the physicists’ appetite for the really heavy stuff. In 1990, in collaboration with several other European laboratories, the construction of a new Linac 3 for the acceleration of lead ions began. It was completed and running in 1994. In the same year, lead ions were accelerated through the PS and SPS.
In the Low-Energy Antiproton Ring (LEAR), oxygen ions were stored and cooled, using both stochastic and electron cooling. Because, for lead–lead collisions, the LHC requires denser beams than the ion source and Linac 3 can provide, the use of LEAR was proposed. Between 1995 and 1997 extensive tests of a scheme for the accumulation and cooling of lead ions in LEAR gave convincing results.
For CERN’s antiproton programme, the PS became involved very early as a major contributor to the Initial Cooling Experiment to test stochastic and electron cooling. In 1978, the decision to go ahead with the Antiproton Project brought the Antiproton Accumulator (AA) to the PS. Apart from the construction of the AA, the PS had to prepare intense proton beams for antiproton production.
The AA started up in 1980, and in 1981 antiprotons were sent to the ISR and soon afterwards to the SPS (which was converted into a proton–antiproton collider) leading to the discovery of W and Z bosons. To increase antiproton production by an order of magnitude, an Antiproton Collector (AC) complemented the AA from 1987.
Once the AA was proven, LEAR was launched for completion in 1982. It received antiprotons from the AA via the PS, which were decelerated there to 600 MeV/c. This was a feat of beam handling. LEAR functioned as a “beam stretcher”. After storing, cooling, decelerating and then cooling again, its antiprotons were fed to the experiments via “ultraslow extraction”. Some 5 x 109 antiprotons were served over periods lasting hours, on average at only one antiproton per revolution.
When antiproton physics finished at the SPS, AC–AA and LEAR continued to provide antiprotons to a rich field of physics until 1996. The AA was dismantled, but the AC is making a comeback as an Antiproton Decelerator (AD). It was first tested with protons in 1998. In 1999 the first antiprotons at 100 MeV/c (5 MeV kinetic energy) are scheduled for new experiments.
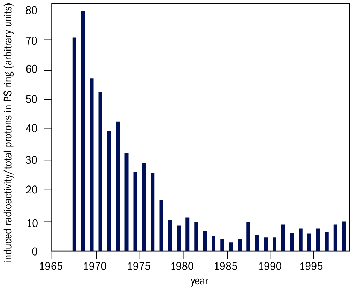
Electrons and positrons
When CERN’s LEP electron–positron collider was conceived, history was repeated. Rather than building new injectors, the PS and SPS could take on the role. More machines had to be added to the PS Complex: a 200 MeV high-intensity electron Linac (to produce positrons from a converter target), a 600 MeV low-intensity electron–positron Linac and an Electron–Positron Accumulator (EPA), from which particles were passed to the PS, accelerated to 3.5 GeV, sent to the SPS for further acceleration and then on to LEP.
For the electron–positron linacs, expertise and help were sought from LAL, Orsay. Construction began in 1984. By 1987 electron and positron beams were ready. Routine LEP operation began in 1989.
Far-reaching modifications had to be made to the PS – the vacuum chamber was entirely changed, electron and positron transfer lines and injections were added, new 114 MHz cavities and three “wigglers” were added too.

The PS Complex today
As well as being a supplier of protons, antiprotons, electrons, positrons and ions to the SPS, the PS Complex has gathered a number of users of its own – protons supplied to the East Hall feed a major experiment (DIRAC) and a number of smaller ones, mostly to test detectors for the LHC experiments. This is also where key experiments for the Energy Amplifier were recently carried out.
ISOLDE moved to the Booster after the closure of the 600 MeV synchrocyclotron in 1990. It is enjoying the higher energy of 1 or 1.4 GeV and intensities of 3 x 1013 protons per pulse. Detector development profits from electrons in an experimental area connected to LIL and EPA. Experiments around the new AD are being readied for 100 MeV/c antiprotons. A new time-of-flight (TOF) facility, using intense proton beams from the PS to produce spallation neutrons, is nearing completion.
The future
For the future, the PS base-load will be the steady delivery of beams to the many clients and maintaining the machines in a good operational state. However, many other tasks are in the offing.
Once the TOF experiment is completed, others, which are using the intense beams from the PS, may follow. The preparation of proton beams to fulfil all of the demands of the LHC requires more effort. There is the conversion of LEAR, for the moment mothballed, to become the Low-Energy Ion Ring, in which lead ions will be accumulated and cooled to the levels required by the LHC. This is a demanding task, which will draw on all the expertise that the PS Division can muster.
The future also means looking beyond the LHC. The vast experience accumulated with a great variety of techniques, machines and beams in the PS Complex is fertile ground. The Compact Linear Collider study has found its home at the PS, with a succession of test facilities (CTF1, CTF2 and now CTF3 is under construction). This will be a major responsibility. A further long-term study has been launched on high-intensity proton accelerators for neutrino factories and, further ahead, a muon collider.
When celebrating a 40th anniversary, it is natural to recall successes and achievements and gloss over thunderstorms, fires, floods and sabotage. Some say that the good old PS should be replaced by something new and better. What is really left of the “good old PS” after all of these upheavals? The astonishing answer is: only the tunnel and 99 of its 100 magnets. Everything else is new, so it really is a “good young” PS. Reserve 24 November 2009 for the PS Golden Jubilee.