Abideh Jafari describes how larger datasets, upgraded detectors and novel analysis methods will allow the Standard Model to be scrutinised at unprecedented levels of precision during Run 3.
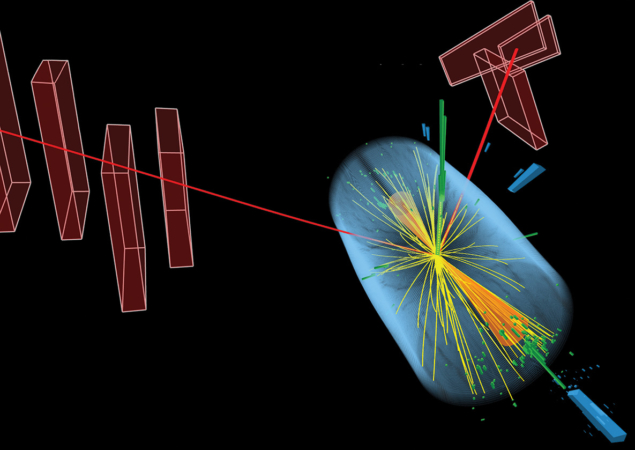
Confronted with multiple questions about how nature works at the smallest scales, we exploit precise measurements of the Standard Model (SM) to seek possible answers. Those answers could further confirm the SM or give hints of new phenomena. As a hadron collider, the LHC was primarily built as a discovery machine. After more than a decade of operation, however, it has surpassed expectations. Alongside the discovery of the Higgs boson and a broad programme of direct searches for new phenomena, ultra-precise measurements on a wide range of parameters have been carried out. These include particle masses, the width of the Z boson and the production cross-sections of various SM processes ranging over 10 orders of magnitude (see “Cross sections” figure); the latter are connected to a multitude of measurements including differential distributions and particle properties.
An example that is unique to the LHC is the measurement of the Higgs-boson mass, which was determined to a precision of 0.12% by CMS in 2019. Also of vital importance are the strengths of the Higgs-boson couplings to other known particles (see “Coupling strengths” figure). According to the SM, these couplings must be proportional to a particle’s mass. Nicely following the SM expectation, every coupling in this plot is extracted using various measurements of the Higgs-boson production and decay channels. Besides the remarkable agreement with the SM, the plot shows the result of the Higgs-boson decay to muons, which is challenging to measure because of the muon’s small mass.
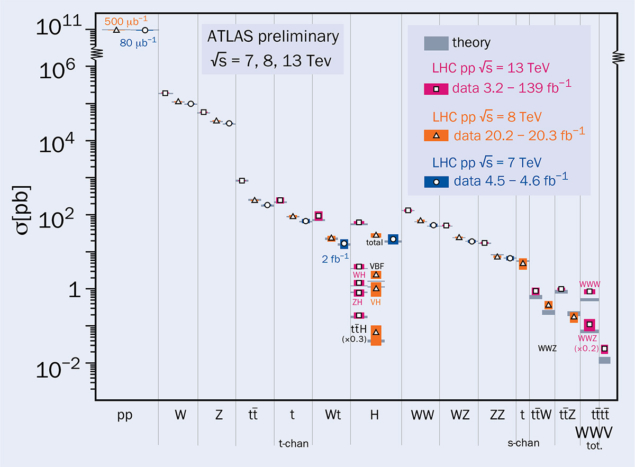
The LHC-experiment collaborations are currently concluding their Run 2 measurements using proton-collision data recorded at 13 TeV while getting ready for the Run 3 startup. From several notable achievements with the Run 2 data, one can point to the measurement of a fundamental parameter of the SM, the mass of the W boson with a precision of 0.02% by ATLAS and of 0.04% in the forward region by LHCb (see “W mass” figure). Precision measurements of the W-boson mass are crucial for testing the consistency of the SM, as radiative corrections connect it with the masses of the top quark and the Higgs boson. A future combination of the LHCb result with similar measurements from ATLAS and CMS can reduce the significant uncertainty of parton distribution functions on this parameter. Although the particle masses are crucial elements of the SM, it is not always possible to determine them directly. In the case of quarks, except for the heaviest top quark, their immediate hadronisation makes the properties of a bare quark inaccessible. Observed for the first time by ALICE, the QCD “dead cone” (an angular region of suppressed gluon emissions surrounding a heavy quark that is proportional to the quark’s mass) in charmed jets may be a possible way to ultimately access the heavy-quark mass directly.
The coupling structure of the SM, especially between heavy particles, is another key aspect that is being pinned down by ATLAS and CMS. In 2017 the experiments marked an important milestone in this regard with the observation of WW scattering – a first step in a diverse programme of measurements of vector boson scattering (VBS), in which vector bosons emitted from each of the incoming quarks interact with one other (see “Critical physics” image). As VBS processes are sensitive to the self-interaction of four gauge bosons as well as to the exchange of a virtual Higgs boson, they remain a central part of the LHC physics programme during Run 3 and beyond, where the additional data will become a decisive factor.
Run 3 preparations
The LHC is about to start a new endeavour at an unprecedented energy (13.6 TeV as opposed to 13 TeV) and with an instantaneous luminosity on average 1.5 times higher than in Run 2. In addition to higher statistics, the larger energy reach of Run 3 provides a unique opportunity to study unexplored territories in the kinematic phase space of particles. Prime targets are regions where the discovery of possible new phenomena is mainly awaiting additional data, and those where the insufficient size of the data sample is the main limiting factor on the precision.

A major challenge ahead is the increased number of additional interactions within the same or nearby bunch crossings, called pileup. The large rate of interactions puts strain on different parts of the detectors as well as their trigger systems. Relying on cutting-edge technologies, experiments at the LHC have performed extensive upgrades in several subsystems, hardware and software to cope with the associated complexities and exploit the full potential of the data. In some cases, this has involved the installation of new detectors or an entire renewal, or extension, of existing subdetectors. Examples are the New Small Wheel (NSW) muon detector in ATLAS and the muon gas electron multiplier (GEM) detectors in CMS. These gas-based detectors, which are designed in view of the High-Luminosity LHC (HL-LHC) and will be partially operational during Run 3, are installed in the endcap area of the experiments where a significant increase is expected in the particle flux. The improved muon momentum resolution they bring also plays a critical role in the trigger systems by keeping the rate low.
It is now proven that with advanced analysis strategies we can surpass the expectations from projection studies
In the ALICE experiment, among other important upgrades, the inner tracker system has faced a complete renewal of the silicon-based detectors for enhanced low-momentum vertexing and tracking capabilities. At LHCb, in addition to new front-end electronics for higher-rate triggering and readout, the ring-imaging Cherenkov detector has been upgraded to deal with the large-pileup environment, while a brand new vertex locator and tracking system will allow the reconstruction of charged particles. In parallel to the hardware, the LHC experiments have accomplished a substantial upgrade in software and computing, including the implementation of fast readout systems and the use of state-of-the-art graphics processing units.
Physics ahead
The series of upgrades undertaken during Long Shutdown 2 will enable the experiments to pursue a rich physics programme during Run 3 and to get ready for Run 4 at the HL-LHC. The preparation also involves Monte Carlo event generation at the new centre-of-mass energy, full simulation of collision events in the new detectors, and designing new methods with modern tools to identify particles and analyse the data. The additional data of Run 3, together with innovative analysis techniques, will result in reduced uncertainties and therefore push the precision frontier forward. The experience from Run 2 is of great value in this regard.
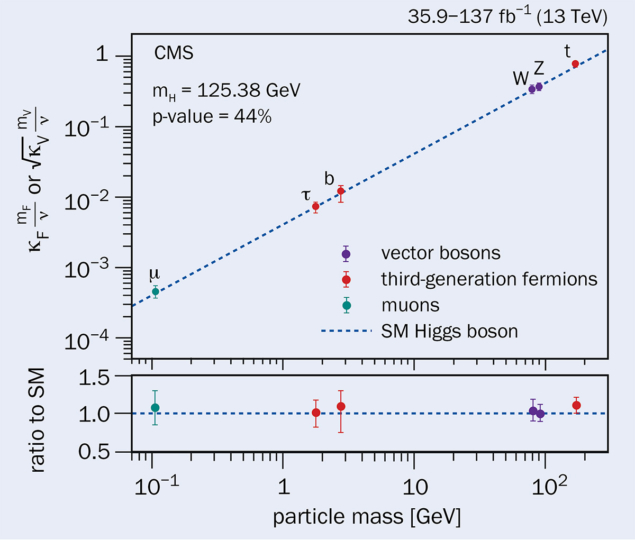
It is now proven that with advanced analysis strategies which make maximal use of the available data, we can surpass the expectations from projection studies. An example is the Higgs-boson decay to muons. Whereas early Run 3 projections suggested an uncertainty of about 20% with 300 fb–1 of LHC data, in 2020 the CMS experiment achieved such precision using Run 2 data alone. In the latest projections, a further improvement of 30–35% is expected thanks to the advanced analysis strategies developed during Run 2. The projected uncertainties in Higgs-boson couplings to other SM particles, including vector bosons and third-generation leptons, are also expected to be reduced. The Higgs-boson interaction with the heaviest known particle, the top quark, is of particular interest as it may give insights into the existence and energy scale of new physics above 100 GeV. Besides the famous ttH process, simultaneous production of four top quarks is also very sensitive to the top quark’s Yukawa interaction with the Higgs boson. Exhibiting the heaviest SM final state, “four-top” is one of the rarest but most important processes. Following evidence reported by ATLAS in 2021, Run 3 data may fully establish its observation.
Among rare processes that may shed light on electroweak symmetry breaking, one can point to the VBS production of longitudinally polarised W bosons. The longitudinal polarisation is a result of electroweak symmetry breaking through which vector bosons acquire mass from their interaction with the Brout–Englert–Higgs field. Given that the analysis of Run 2 data has reached the expected significance (about 1σ) of the HL-LHC with the same luminosity, we look forward to Run 3 to test the SM with more data and further channels.
Run 3 excitement
The excitement about LHC Run 3 is not restricted to rare phenomena and new discoveries. Well-established processes such as top-quark, W- and Z-boson production are pivotal for a firm understanding of the SM. The upcoming data will provide us with gigantic statistics that translates to a significantly higher precision on the measured properties of these particles in addition to various fundamental parameters of the SM. The latter include the mass of the top quark, the precise determination of which is a critical factor in the stability of vacuum. Early Run 3 projection studies predicted an uncertainty of 1.5 GeV on the top-quark mass. This has already been achieved in Run 2 using tt differential cross-section measurements, and will be further reduced with the upcoming Run 3 data.
The upcoming data will provide us with gigantic statistics that translate to significantly higher precision
Such levels of precision also provide invaluable feedback to the theory community, whose tremendous efforts in modelling and state-of-the-art calculations and simulations are the basis of our measurements. Thanks to the increasing sophistication and precision of SM calculations, any statistically significant deviation from theory can be an unambiguous sign of new physics. Therefore, precision measurements in Run 3 can act as a gateway to new discoveries. These include measurements of properties such as vector-boson polarisation, which are sensitive to new physics by construction, inclusive cross sections of VBS and other rare processes, and differential distributions where new phenomena can appear in the tails.
In October 2021, stable proton beams were circulated and collided at a centre-of-mass energy of 900 GeV in the LHC for the first time since 2018. While preparing for the start up in May this year, the experiments made use of these data for a special period of commissioning to ensure their readiness to collect data in Run 3. The successful outcome of the commissioning brought further enthusiasm and motivation to the LHC-experiment collaborations, who very much look forward to executing their far-reaching Run 3 physics plans.
To explore all our coverage marking the 10th anniversary of the discovery of the Higgs boson ...