Rama Calaga describes the latest progress in building the superconducting radio-frequency “crab” cavities needed to maximise the scientific output
of the High-Luminosity LHC.
The imminent start of LHC Run 3 following a vast programme of works completed during Long Shutdown 2 marks a milestone for the CERN accelerator complex. When stable proton beams return to the LHC this year (see LHC Run 3: the final countdown), they will collide at higher energies (13.6 compared to 13 TeV) and with higher luminosities (containing up to 1.8 × 1011 protons per bunch compared to 1.3–1.4 × 1011) than in Run 2. Physicists working on the LHC experiments can therefore look forward to a rich harvest of results during the next three years. After Run 3, the statistical gain in running the accelerator without a significant luminosity increase beyond its design and ultimate values will become marginal. Therefore, to maintain scientific progress and to exploit its full capacity, the LHC is undergoing upgrades that will allow a decisive increase of its luminosity during Run 4, expected to begin in 2029, and beyond.
Several technologies are being developed for this High-Luminosity LHC (HL-LHC) upgrade. One is new, large-aperture quadrupole magnets based on a niobium-tin superconductor. These will be installed on either side of the ATLAS and CMS experiments, providing the space required for smaller beam-spot sizes at the interaction points and shielding against the higher radiation levels when operating at increased luminosities. The other key technology, necessary to take advantage of the smaller beam-spot size at the interaction points, is a series of superconducting radio-frequency (RF) “crab” cavities that enlarge the overlap area of the incoming bunches and thus increase the probability of collisions. Never used before at a hadron collider, a total of 16 compact crab cavities will be installed on either side of each of ATLAS and CMS once Run 3 ends and Long Shutdown 3 begins.
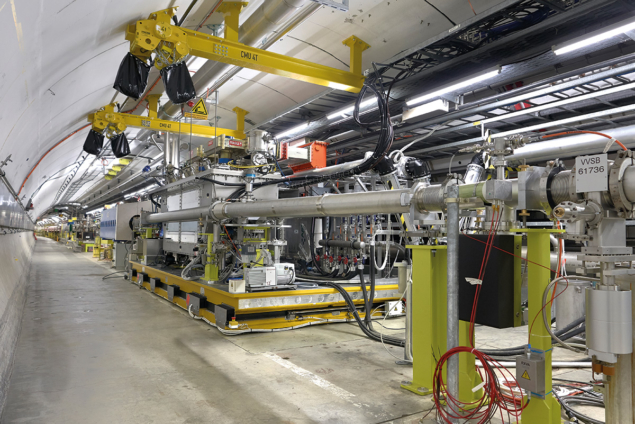
At a collider such as the LHC, it is imperative that the two counter-circulating beams are physically separated by an angle, aka the crossing angle, such that bunches collide only in one single location over the common interaction region (where the two beams share the same beam pipe). The bunches at the HL-LHC will be 10 cm long and only 7 μm wide at the collision points, resembling thin long wires. As a result, even a very small angle between the bunches implies an immediate loss in luminosity. With the use of powerful superconducting crab cavities, the tilt of the bunches at the collision point can be precisely controlled to make it optimal for the experiments and fully exploit the scientific potential of the HL-LHC.
Radical concepts
The tight space constraints from the relatively small separation of the two beams outside the common interaction region requires a radically new RF concept for particle deflection, employing a novel shape and significantly smaller cavities than those used in other accelerators. Designs for such devices began around 10 years ago, with CERN settling on two types: double quarter wave (DQW) and RF-dipole (RFD). The former will be fitted around CMS, where bunches are separated vertically, and the latter around ATLAS, where bunches will be separated horizontally, requiring crab cavities uniquely designed for each plane. It is also planned to swap the crossing-angle planes and crab-cavity installations at a later stage during the HL-LHC operation.

In 2017, two prototype DQW-type cavities were built and assembled at CERN into a special cryomodule and tested at 2 K, validating the mechanical, cryogenic and RF functioning. The module was then installed in the Super Proton Synchrotron (SPS) for beam tests, with the world’s first “crabbing” of a proton beam demonstrated on 31 May 2018. In parallel, the fabrication of two prototype RFD-type cavities from high-purity niobium was underway at CERN. Following the integration of the devices into a titanium helium tank at the beginning of 2021, and successful tests at 2 K reaching voltages well beyond the nominal value of 3.4 MV, the cavities were equipped with specially designed RF couplers, which are necessary for beam operations. The two cavities are now being integrated into a cryomodule at Daresbury Laboratory in the UK as a joint effort between CERN and the UK’s Science and Technology Facilities Council (STFC). The cryomodule will be installed in a 15 m-long straight section (LSS6) of the SPS in 2023 for its first test with proton beams. This location in the SPS is equipped with a special by-pass and other services, which were put in place in 2017–2018 to test and operate the DQW-type module.
The manufacturing challenge
Due to the complex shape and micrometric tolerances required for the HL-LHC crab cavities, a detailed study was performed to realise the final shape through forming, machining, welding and brazing operations on the high-purity niobium sheets and associated materials (see “Fine machining” images). To ensure a uniform removal of material along the cavities’ complex shape, a rotational buffer chemical polishing (BCP) facility was built at CERN for surface etching of the HL-LHC crab cavities. For the RFD and DQW, the rotational setup etches approximately 250 μm of the internal RF surface to remove the damaged cortical layer during the forming process. Ultrasound measurements were performed to follow the evolution of the cavity-wall thickness during the BCP steps, showing remarkable uniformity (see “Chemical etching” images).
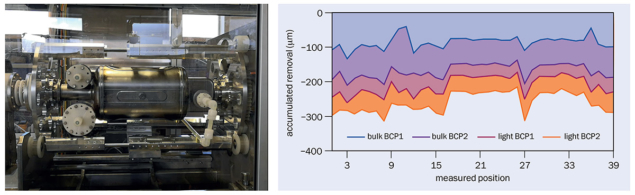
Preparation of the RFD cavities involved a similar process as that for the DQW modules. Following chemical etching and a very high-temperature bake at 650 °C in a vacuum furnace, the cavities are rinsed in ultra-pure water at high pressure (100 bar) for approximately seven hours. This process has proven to be a key step in the HL-LHC crab-cavity preparation to enable extremely high fields and suppress electron-field emitters, which can limit the performance. The cavity is then closed with its RF ancillaries in an ISO4 cleanroom environment to preserve the ultra-clean RF surface, and installed into a special vertical cryostat to cool the cavity surface to its 2 K operating temperature (see “Clean and cool” image, top). Both RFD cavities reached performances well above the nominal target of 3.4 MV. RFD1 reached more than 50% over the nominal voltage and RFD2 reached above a factor of two (7 MV) – a world-record deflecting field in this frequency range. These performances were reproducible after the assembly and welding of the helium tank owing to the careful preparation of the RF surface throughout the different steps of assembly and preparation.

The helium tank provides a volume around the cavity surface that is maintained at 2 K with superfluid helium (see “Clean and cool” image, bottom). Due to sizeable deformations during the cool-down process from ambient temperature, a titanium vessel which has a thermal behaviour close to that of the niobium cavity is used. A magnetic shield between the cavity and the helium tank suppresses stray fields in the operating environment and further preserves cavity performance. Following the tests with helium tanks, the cavities were equipped with higher-order-mode couplers and field antennae to undergo a final test at 2 K before cryostating them into a two-cavity string.
The crab cavities require many ancillary components to allow them to function. This overall system is known as a cryomodule (see “Cryomodule” image, top) and ensures that the operational environment is correct, including the temperature, stability, vacuum conditions and RF frequency of the cavities. Technical challenges arise due to the need to assemble the cavity string in an ISO4 cleanroom, the space constraints of the LHC (leading to the rectangular compact shape), and the requirement of fully welded joints (where typically “O” rings would be used for the insulation vacuum).
Design components
The outer vacuum chamber (OVC) of the cryomodule provides an insulation vacuum to prevent heat leaking to the environment as well as providing interfaces to any external connections. Manufactured by ALCA Technology in Italy, the OVC used a rectangular design where the cavity string is mounted to a top-plate that is lowered into the rest of the OVC, and includes four large windows to allow access for repair in situ if required (see “Cryomodule” image, bottom). Since the first DQW prototype module, several cryomodule interfaces including cryogenic and vacuum components were updated to be fully compatible with the final installation in the HL-LHC.
The HL-LHC crab-cavity programme has developed into a mature project supported by a large number of collaborating institutions around the world
Since superconducting RF cavities can have a higher surface resistance if cooled below their transition temperature in the presence of a magnetic field, they need to be shielded from Earth’s magnetic field and stray fields in the surrounding environment. This is achieved using a warm magnetic shield manufactured in the OVC, and a cold magnetic shield mounted inside the liquid-helium vessel. Both shields, which are made from special nickel-iron alloys, are manufactured by Magnetic Shields Ltd in the UK.
Status and outlook
The RFD crab-cavity pre-series cryomodule will be assembled this year at Daresbury lab, where the infrastructure on site has been upgraded, including an extension to the ISO4 cleanroom area and the introduction of an ISO6 preparation area. A bespoke five-tonne crane has also been installed and commissioned to allow the precise lowering of the delicate cavity string into the outer vacuum vessel.
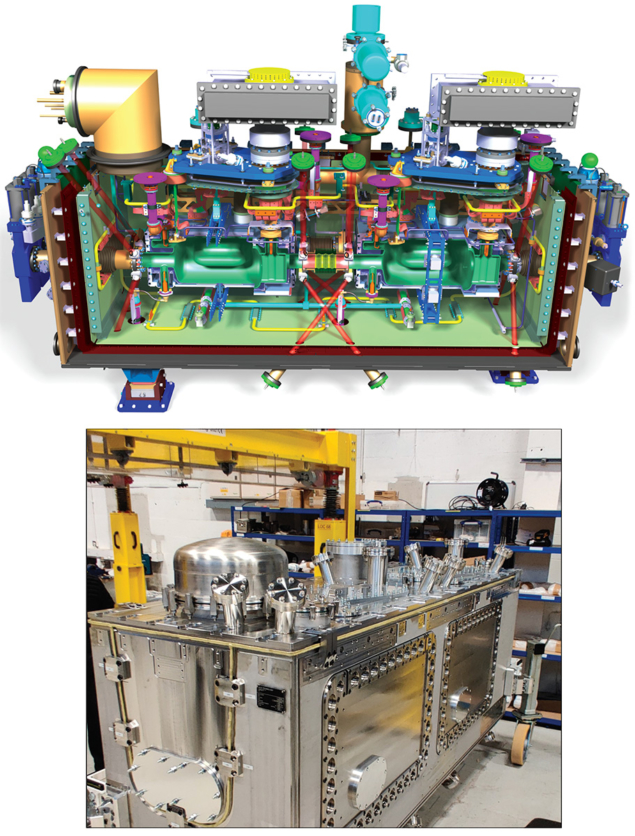
Parallel activities are taking place elsewhere. The HL-LHC crab-cavity programme has developed into a mature project supported by a large number of collaborating institutions around the world. In the US, the Department of Energy is supporting the HL-LHC Accelerator Upgrade Project to coordinate the efforts and leverage the expertise of a group of US laboratories and universities (FNAL, BNL, JLAB, SLAC, ODU) to deliver the series RFD cavities for the HL-LHC. In 2021, two RFD prototype cavities were built by the US collaboration and exceeded the two most important functional project requirements for crab cavities – deflecting voltage and quality factor. After this successful demonstration, the fabrication of the pre-series cavities was launched.
Crab cavities were first implemented in an accelerator in 2006, at the KEKB electron–positron collider in Japan, where they helped the collider reach record luminosities. A different “crab-waist” scheme is currently employed at KEKB’s successor, SuperKEKB, helping to reach even higher luminosities. The development of ultra-compact, very-high-field cavities for a high-energy hadron collider such as the HL-LHC is even more challenging, and will be essential to maximise the scientific output of this flagship facility beyond the 2030s.
Beyond the HL-LHC, the compact crab-cavity concepts have been adopted by future facilities, including the proton–proton stage of the proposed Future Circular Collider; the Electron–Ion Collider under construction at Brookhaven; bunch compression in synchrotron X-ray sources to produce shorter pulses; and ultrafast particle separators in proton linacs to separate bunches of secondary particles for different experiments. The full implementation of this technology at the HL-LHC is therefore keenly awaited.