The science of neutrino physics has reached a watershed, with discovery giving way to precision measurements. Michael Altmann reports from the XXth International Conference on Neutrino Physics and Astrophysics.
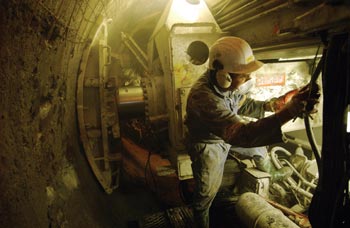
“We return from this conference in the firm belief that this novel phenomenon indeed does occur,” Friedrich Dydak of CERN told a journalist following the XXth International Conference on Neutrino Physics and Astrophysics (Neutrino 2002), held at Munich Technical University. He was referring to neutrino oscillations, the periodic transformation between neutrinos of different flavour.
The Sudbury Neutrino Observatory’s (SNO) confirmation of oscillations was a highlight in an unprecedented wealth of fundamentally important new results. These results, along with their far-reaching implications, attracted some 450 scientists from all over the world to attend Neutrino 2002. The conference, held on 24-30 May, was jointly organized by Munich Technical University and the Max Planck Institute of Physics (MPI). Presentations and discussions underlined neutrino physics as currently being among the most exciting and rewarding fields of research.
With the discoveries of neutrino masses and lepton-flavour mixing having been established in recent years in studies of solar and atmospheric neutrinos, the field is currently in the transition to a new era. There is a general consensus that the next natural step is precision studies of the neutrinos’ intrinsic parameters. This will put additional emphasis on terrestrial experiments. However, neutrino astronomy is also rapidly evolving – neutrinos are being used as unique tools for astrophysical observations, promising insights into long-standing mysteries such as the origin and acceleration mechanisms of ultra-high-energy cosmic rays.
The conference started with SNO’s confirmation of neutrino oscillations in their measurement of solar boron-8 neutrinos, detailed by Aksel Hallin of Queen’s University, Canada. Neutrino interactions in SNO’s 1000 tonne heavy-water target allow separate determination of the fluxes of solar electron neutrinos (F(ne)), and of all active flavours together (F(ne) + (F(nm) + (F(nt)). While determination of the latter shows perfect agreement with predictions from solar model computations, a substantial ne deficit is observed. This constitutes the first direct observation of neutrino flavour transition, thus confirming the indirect evidence gathered by earlier solar neutrino experiments. Among the processes that could account for the observed phenomena, neutrino oscillation is the favoured explanation. Taking the SNO result together with those of the GALLEX/GNO experiment at Italy’s Gran Sasso laboratory; the Russian-American SAGE experiment; Japan’s Superkamiokande; and the pioneer of them all, Ray Davis’ experiment in the Homestake mine (all of which presented their latest results), possible values for the neutrino mass squared difference Dm2 and mixing angle tan2f can be restricted to a few well defined areas.
Global analyses tend to favour the so-called large mixing angle (LMA) region with parameters in the range 3 ¥ 10-5 eV2 £ Dm2 £ 3 ¥ 10-4 eV2 and 0.25 £ tan2f £ 0.8. This region, which was first identified as a possible solution to the solar neutrino puzzle in 1992 with the first results from GALLEX, offers an attractive feature – it can be tested and further scrutinized by entirely terrestrial experiments. A key role will be played by the Japanese experiment KamLAND, which aims to detect electron antineutrinos emitted by nuclear power reactors situated within distances of a few hundred kilometres.
Low-energy neutrinos

For parameters in the LMA region, neutrino oscillations will lead to a substantial, energy-dependent suppression of the recorded electron antineutrino flux. First data from KamLAND are expected this autumn. However, KamLAND is not particularly sensitive in investigating the high Dm2 part of the LMA region. To achieve this goal, a substantially shorter baseline of around 20 km would be necessary. Stefan Schönert of MPI Heidelberg presented such a concept in his talk on future projects in the field of low-energy oscillation physics.
Schönert also gave a summary of the Third International Workshop on Low Energy Solar Neutrinos (LowNu 2002), which had taken place at Heidelberg as a topical satellite meeting prior to the conference. With an attendance of about 80, and some 30 presentations comprehensively covering ongoing activities, the workshop focused on experimental projects, common techniques and challenges, as well as future physics impact. It identified as the main goal for the forthcoming years the exploration of the primary pp, berylium-7, pep, and, if possible, the CNO solar neutrino fluxes, in real time and with energy information via both elastic ne scattering (es) and charged-current (cc) interaction. With its measurements of high-energy boron-8 neutrinos, SNO has already pioneered such techniques and impressively illustrated their potential for determining intrinsic neutrino parameters and testing our understanding of stellar energy-generation mechanisms. Extending the es versus cc measurement to the medium and low-energy branches of the solar neutrino spectrum is yielding increased precision for both these issues. A first step will be the es measurement of solar berylium-7 neutrinos in the BOREXINO experiment, currently being prepared at Gran Sasso.
Atmospheric neutrinos
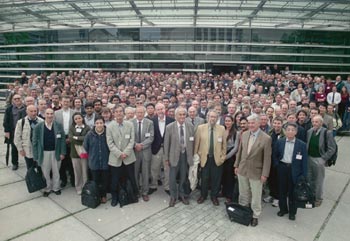
At the 1998 neutrino conference, the Superkamiokande collaboration reported evidence for oscillations of neutrinos produced by the interaction of cosmic rays with the Earth’s atmosphere. This year, Masato Shiozawa of the University of Tokyo presented a new analysis comprising about five years of data. The collaboration has identified tau-like events at multi-GeV energies, supporting the channel nm to nt as the predominant oscillation mode of atmospheric muon neutrinos. Moreover, oscillations into a hypothetical sterile neutrino are strongly disfavoured by this analysis. Superkamiokande’s preferred parameter range is 1.6 x 10-3 eV2 £ Dm2 £ 3.9 x 10-3 at essentially maximal mixing.
Though the analysis of atmospheric neutrino data generally relies on a ratio determination of electron to muon neutrinos, and is therefore rather insensitive to uncertainties in the absolute fluxes, a reliable understanding of neutrino generation in the atmosphere is of interest nonetheless. Thomas Gaisser of the University of Delaware reported the substantial progress achieved over recent years in modelling the processes involved. Meanwhile, the first reliable 3-D computations have become available. Among other phenomena, which still have to be incorporated into the analysis of the experimental data, predictions for the high-energy range above around 100 GeV will turn out to be useful for calibrating future neutrino telescopes.
As for solar neutrinos, the parameters giving rise to oscillations of atmospheric neutrinos are accessible for test in terrestrial experiments due to the LMA. In the KEK to Kamioka (K2K) project, a neutrino beam produced at Japan’s KEK proton synchrotron is directed over a distance of 250 km towards the Superkamiokande detector. For the currently available data set (recorded before the detector’s devastating accident of November last year) some 80 events were expected in the absence of oscillations, whereas only 56 events were observed. This number is in good agreement with the neutrino parameter set favoured by Superkamiokande’s atmospheric neutrino measurements.
Future long-baseline experiments
To further substantiate the evidence for oscillations gathered with atmospheric neutrinos, future experiments are aimed at directly mapping the oscillatory signature and at performing tau appearance studies. Two new projects are currently being prepared in the US and Europe to further investigate the parameter region favoured by current data. Both are situated at a baseline of 732 km. The American MINOS detector in Minnesota’s Soudan mine will receive a muon neutrino beam from Fermilab, whereas the European CERN neutrinos to Gran Sasso (CNGS) project will send a beam from CERN to the ICARUS and OPERA detectors, to be installed at Gran Sasso.
In contrast to K2K, which operates at an energy below the tau production threshold, these new projects will use beams of much higher energy. MINOS is a massive 5.4 kt steel/scintillator calorimeter, whereas the ICARUS collaboration chose a novel approach with their liquid argon Time Projection Chamber. OPERA will identify taus in a large-scale sandwiched lead/emulsion detector. MINOS is scheduled to start data-taking by 2005. OPERA and ICARUS will be operational from 2006.
These projects are only the next step in accelerator-based long-baseline studies.
However, these projects are only the next step in accelerator-based long-baseline studies. Plans for much more ambitious next-to-next-generation beams and detectors in Japan, Europe and the US were presented and extensively discussed. Such superbeams, and ultimately a neutrino factory, will open the door to precision physics with accelerator-produced neutrinos. If LMA is indeed the parameter region realized in nature, a determination of Dm2 and of elements of the lepton-mixing matrix to an accuracy in the few percent range seems feasible. Even matter effects and leptonic charge-parity (CP) violation could be experimentally accessible. However, the challenges for producing neutrino beams with the required intensity and quality are enormous, and in view of the technical complexity it is probably not unrealistic to estimate the need for at least another decade of R&D before a neutrino factory can be built.
Current accelerator experiments
The conclusions to be drawn from the current short-baseline accelerator-based experiments are still unclear. On one hand, the positive effect detected by the Los Alamos LSND experiment in its search for neutrino oscillations persists, whereas the Karlsruhe-Rutherford Laboratory experiment KARMEN sees no indication for oscillations. However, KARMEN cannot exclude the entire parameter space favoured by LSND, so the final answer will have to wait for Fermilab’s MiniBoone experiment (MiniBOONE goes live at Fermilab). The result from MiniBoone is eagerly awaited, particularly because it could be decisive for the fundamental question of whether a fourth neutrino flavour exists. If LSND is correct, the existence of such a fourth generation, which has to be sterile with respect to weak interaction, is an inevitable consequence.
Intrinsic neutrino properties
Neutrino oscillations imply that neutrinos are massive. However, oscillation experiments only give information on the mass difference, not on the mass eigenvalues themselves. Knowledge of the absolute masses is crucial for judging the role neutrinos play in astrophysical processes and cosmology. A degenerate neutrino mass scale in the few-eV range, for example, would imply that neutrinos contribute substantially to the mass of the universe. Absolute neutrino masses can be tested by direct kinematical methods. With the present Mainz and Troitsk experiments (which investigate the spectral shape of tritium decay close to the endpoint) having reached their sensitivity limit of 2.2 eV for the mass of the electron neutrino, the large-scale Karlsruhe tritium neutrino experiment KATRIN project was put forward as a follow-up. KATRIN will start data-taking in 2007, and intends to achieve sub-eV sensitivity.
Another long-standing fundamental question is whether neutrinos are of Majorana type (their own antiparticles). A recent claim of possible evidence for neutrinoless double-beta decay, a phenomenon that can occur only for Majorana-type neutrinos, has attracted considerable attention. Following a presentation of Oliviero Cremonesi from Milan University, who reviewed the various experiments, there was a vigorous discussion on the statistical significance and trustworthiness of the analysis leading to the claimed evidence. No consensus has yet been reached, and it remains for future experiments and improved statistics to settle this question.
Neutrinos in astrophysics and cosmology
Massive neutrinos play an important role during core collapse supernova explosions, as John Beacom of Fermilab pointed out. However, both the energy released in the form of neutrinos of the second and third flavour and their mean temperature are still uncertain. They have not yet been measured, and the models depend on many assumptions. The new KamLAND and BOREXINO scintillator detectors, however, can determine these parameters to an accuracy of 10%, thereby offering the potential to substantially improve our knowledge of supernova physics.
Precision measurements of the large-scale structure of our universe and the cosmic microwave background radiation can be used to probe intrinsic neutrino properties, most notably absolute neutrino masses and the hypothesis of sterile neutrinos. Combining cosmic microwave background data from NASA’s MAP and ESA’s Planck missions, and the Sloan Digital Sky Survey, should allow a limit of under 0.3 eV to be established – a sensitivity comparable to that of the terrestrial KATRIN experiment.
Cosmology sometimes teaches us lessons about neutrino properties, but sometimes the inverse is also true. Tsutomu Yanagida of Tokyo showed how massive neutrinos could lead to baryon asymmetry by means of leptogenesis. Inevitable consequences of the proposed mechanism, however, are neutrinoless double-beta decay and leptonic CP violation. Steve King of Southampton pointed out that these two phenomena also turn out to be decisive among the many different models proposed to explain neutrino masses (and their obvious smallness) and mixing angles.
Many of the theories beyond the Standard Model not only explain massive neutrinos, but also predict additional, exotic particles that constitute appealing candidates to contribute to the universe’s dark matter. A prime candidate among them is non-relativistic weakly interacting massive particles (WIMPs) such as the neutralino. Oxford’s Yorck Ramachers presented the large number of experiments searching for WIMPs that are operational or being prepared, along with the different experimental techniques they employ.
For several years the Italian DAMA experiment, a 100 kg sodium iodide detector, has been observing evidence for a WIMP signal, a claim that could have enormous consequences for particle physics, astrophysics and cosmology. Clearly, such a claim needs confirmation by independent experiments. The French EDELWEISS group, which operated a 320 g cryogenic detector, reported that they have now reached a sensitivity comparable to that of DAMA, an achievement made possible by the active background rejection their detector is capable of. However, the EDELWEISS data exclude most of the parameter region favoured by DAMA. With the upgrade phases of the German-British-Italian CRESST experiment, the US CDMS search and EDELWEISS that are currently being installed, the sensitivity region will be expanded by at least two orders of magnitude towards lower coupling strengths within a few years. This will not only give the final answer to whether the DAMA evidence is real, but also probe a substantial part of the parameter region predicted by the most economical supersymmetric extension to the Standard Model (the minimal supersymmetric Standard Model).
Neutrino telescopes
Eli Waxman of Israel’s Weizmann Institute explained that many different objects and processes in the universe are expected to emit high-energy neutrinos, among them gamma-ray bursts, active galactic nuclei and supernova remnants. So far, however, the first-generation neutrino telescope at Lake Baikal and the AMANDA detector at the south pole, limited in sensitivity by their size, have not identified any point sources, but rather posed flux limits. To explore the region up to a distance of 1010 light-years and to investigate the expected diffuse flux of high-energy neutrinos, detector masses in the gigatonne range, corresponding to instrumented volumes of about a cubic kilometre, are required. Experience with Baikal and AMANDA has demonstrated that such huge detectors are feasible. In fact, with the IceCube project in Antarctica and the prototype installations of NESTOR and ANTARES in the Mediterranean, construction of these next-generation detectors has already begun. Undoubtedly, they will open up new avenues in neutrino astronomy.
<textbreak=Outlook>”Where do we stand and where are we going?” This was the question addressed by the two concluding talks. Michel Spiro of Saclay and Boris Kayser of Fermilab summarized in turn the most striking neutrino topics from an experimental and a theoretical perspective. Though the establishment of massive neutrinos is a fundamental breakthrough, many open questions and problems for further experimental and theoretical investigations remain. Mass eigenvalues and mixing angles are still to be determined. The questions of leptonic CP violation, dipole moments, mass hierarchy schemes and decay modes remain open. And the fundamental issue of whether the neutrino is of Dirac or Majorana nature still needs to be resolved. Moreover, there are many new applications of neutrinos as tools and messengers in astronomy, astrophysics and cosmology on the horizon to keep neutrino physics and neutrino astrophysics at the forefront of modern research. We may look forward to new fundamental results and discoveries to be discussed at the next neutrino conference, to be held in Paris in 2004.
Further reading
Transparencies are available at http://neutrino2002.ph.tum.de for Neutrino 2002.