A report from the LHCb experiment.
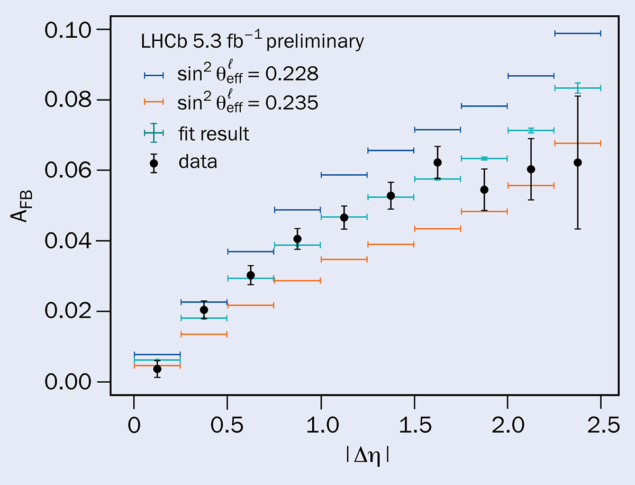
At the International Conference on High-Energy Physics in Prague in July, the LHCb collaboration presented an updated measurement of the weak mixing angle using the data collected at the experiment between 2016 and 2018. The measurement benefits from the unique forward coverage of the LHCb detector.
The success of electroweak theory in describing a wide range of measurements at different experiments is one of the crowning achievements of the Standard Model (SM) of particle physics. It explains electroweak phenomena using a small number of free parameters, allowing precise measurements of different quantities to be compared to each other. This facilitates powerful indirect searches for beyond-the-SM physics. Discrepancies between measurements might imply that new physics influences one process but not another, and global analyses of high-precision electroweak measurements are sensitive to the presence of new particles at multi-TeV scales. In 2022 the entire field was excited by a measurement of the W-boson mass that is significantly larger than the value predicted within these global analyses by the CDF collaboration, heightening interest in electroweak measurements.
The weak mixing angle is at the centre of electroweak physics. It describes the mixing of the U(1) and SU(2) fields, determines couplings of the Z boson, and can also be directly related to the ratio of the W and Z boson masses. Excitingly, the two most precise measurements to date, from LEP and SLD, are in significant tension. This raises the prospect of non-SM particles potentially influencing one of these measurements, since the weak mixing angle, as a fundamental parameter of nature, should otherwise be the same no matter how it is measured. There is therefore a major programme measuring the weak mixing angle at hadron colliders, with important contributions from CDF, D0, ATLAS, CMS and LHCb.
Since the weak mixing angle controls Z-boson couplings, it can be determined from measurements of the angular distributions of Z-boson decays. The LHCb collaboration measured around 860,000 Z-boson decays to two oppositely charged muons, determining the relative rate at which negatively charged muons are produced closer to the LHC beamline than positively charged muons as a function of the angular separation of the two muons. Corrections are then applied for detector effects. Comparison to theoretical predictions based on different values of the weak mixing angle allows the value best describing the data to be determined (figure 1).
The unique angular coverage of the LHCb detector is well-suited for this measurement for two key reasons. First, the statistical sensitivity to the weak mixing angle is largest in the forward region close to the beamline that the LHCb detector covers. Second, the leading systematic uncertainties in measurements of the weak mixing angle at hadron colliders typically arise from existing knowledge of the proton’s internal structure. These uncertainties are also smallest in the forward region.
The value of the weak mixing angle measured by LHCb is consistent with previous measurements and with SM expectations (see “Weak mixing angle” figure). Notably, the precision of the LHCb measurement remains limited by the size of the data sample collected, such that further improvements are expected with the data currently being collected using the upgraded LHCb detector. In addition, while other experiments profile effects associated with the proton’s internal structure to reduce uncertainties, the unique forward acceptance means that this is not yet necessary at LHCb. This advantage will also be important for future measurements: the small theoretical uncertainty means that the forthcoming Upgrade 2 of the LHCb experiment is expected to achieve a precision more than a factor of two better than the most precise measurements to date.