The DUNE experiment is taking shape deep in the same mine where physicists got the first hint that something was amiss with the neutrino.
In 1968, deep underground in the Homestake gold mine in South Dakota, Ray Davis Jr. observed too few electron neutrinos emerging from the Sun. The reason, we now know, is that many had changed flavour in flight, thanks to tiny unforeseen masses.
At the same time, Steven Weinberg and Abdus Salam were carrying out major construction work on what would become the Standard Model of particle physics, building the Higgs mechanism into Sheldon Glashow’s unification of the electromagnetic and weak interactions. The Standard Model is still bulletproof today, with one proven exception: the nonzero neutrino masses for which Davis’s observations were in hindsight the first experimental evidence.
Today, neutrinos are still one of the most promising windows into physics beyond the Standard Model, with the potential to impact many open questions in fundamental science (CERN Courier May/June 2024 p29). One of the most ambitious experiments to study them is currently taking shape in the same gold mine as Davis’s experiment more than half a century before.
Deep underground
In February this year, the international Deep Underground Neutrino Experiment (DUNE) completed the excavation of three enormous caverns 1.5 kilometres below the surface at the new Sanford Underground Research Facility (SURF) in the Homestake mine. 800,000 tonnes of rock have been excavated over two years to reveal an underground campus the size of eight soccer fields, ready to house four 17,500 tonne liquid–argon time-projection chambers (LArTPCs). As part of a diverse scientific programme, the new experiment will tightly constrain the working model of three massive neutrinos, and possibly even disprove it.
DUNE will measure the disappearance of muon neutrinos and the appearance of electron neutrinos over 1300 km and a broad spectrum of energies. Given the long journey of its accelerator-produced neutrinos from the Long Baseline Neutrino Facility (LBNF) at Fermilab in Illinois to SURF in South Dakota, DUNE will be uniquely sensitive to asymmetries between the appearance of electron neutrinos and antineutrinos. One predicted asymmetry will be caused by the presence of electrons and the absence of positrons in the Earth’s crust. This asymmetry will probe neutrino mass ordering – the still unknown ordering of narrow and broad mass splittings between the three tiny neutrino masses. In its first phase of operation, DUNE will definitively establish the neutrino mass ordering regardless of other parameters.
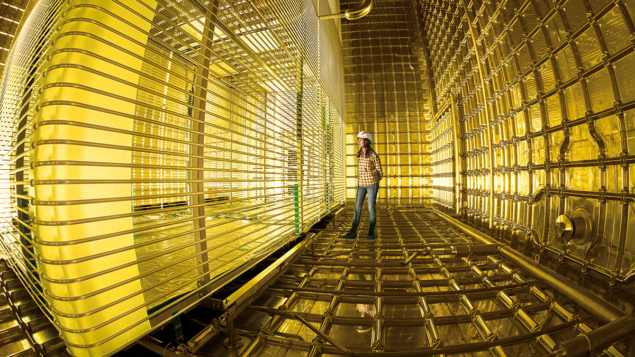
If CP symmetry is violated, DUNE will then observe a second asymmetry between electron neutrinos and antineutrinos, which by experimental design is not degenerate with the first asymmetry. Potentially the first evidence for CP violation by leptons, this measurement will be an important experimental input to the fundamental question of how a matter–antimatter asymmetry developed in the early universe.
If CP violation is near maximal, DUNE will observe it at 3σ (99.7% confidence) in its first phase. In DUNE and LBNF’s recently reconceptualised second phase, which was strongly endorsed by the US Department of Energy’s Particle Physics Project Prioritization Panel (P5) in December (CERN Courier January/February 2024 p7), 3σ sensitivity to CP violation will be extended to more than 75% of possible values of δCP, the complex phase that parameterises this effect in the three-massive-neutrino paradigm.
Combining DUNE’s measurements with those by fellow next-generation experiments JUNO and Hyper-Kamiokande will test the three-flavour paradigm itself. This paradigm rotates three massive neutrinos into the mixtures that interact with charged leptons via the Pontecorvo–Maki–Nakagawa–Sakata (PMNS) matrix, which features three angles in addition to δCP.
As well as promising world-leading resolution on the PMNS angle θ23, DUNE’s measurements of θ13 and the Δm232 mass splitting will be different and complementary to those of JUNO in ways that could be sensitive to new physics. JUNO, which is currently under construction in China, will operate in the vicinity of a flux of lower-energy electron antineutrinos from nuclear reactors. DUNE and Hyper-Kamiokande, which is currently under construction in Japan, will both study accelerator-produced sources of muon neutrinos and antineutrinos, though using radically different baselines, energy spectra and detector designs.
Innovative and impressive
DUNE’s detector technology is innovative and impressive, promising millimetre-scale precision in imaging the interactions of neutrinos from accelerator and astrophysical sources (see “Millimetre precision” image). The argon target provides unique sensitivity to low-energy electron neutrinos from supernova bursts, while the detectors’ imaging capabilities will be pivotal when searching for beyond-the-Standard-Model physics such as dark matter, sterile-neutrino mixing and non-standard neutrino interactions.
First proposed by Nobel laureate Carlo Rubbia in 1977, LArTPC technology demonstrated its effectiveness as a neutrino detector at Gran Sasso’s ICARUS T600 detector more than a decade ago, and also more recently in the MicroBooNE experiment at Fermilab. Fermilab’s short-baseline neutrino programme now includes ICARUS and the new Short Baseline Neutrino Detector, which is due to begin taking neutrino data this year.
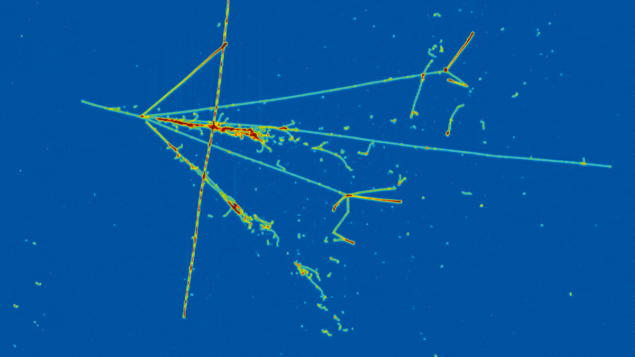
The first phase of DUNE will construct one LArTPC in each of the two detector caverns, with the second phase adding an additional detector in each. A central utility cavern between the north and south caverns will house infrastructure to support the operation of the detectors.
Following excavation by Thyssen Mining, final concrete work was completed in all the underground caverns and drifts, and the installation of power, lighting, plumbing, heating, ventilation and air conditioning is underway. 90% of the subcontracts for the installation of the civil infrastructure have already been awarded, with LBNF and DUNE’s economic impact in Illinois and South Dakota estimated to be $4.3 billion through fiscal years 2022 to 2030.
Once the caverns are prepared, two large membrane cryostats will be installed to house the detectors and their liquid argon. Shipment of material for the first of the two cryostats being provided by CERN is underway, with the first of approximately 2000 components having arrived at SURF in January; the remainder of the steel for the first cryostat was due to have been shipped from its port in Spain by the end of May. The manufacture of the second cryostat by Horta Coslada is ongoing (see “Cryostat creation” image).
Procedures for lifting and manipulating the components will be tested in South Dakota in spring 2025, allowing the collaboration to ensure that it can safely and efficiently handle bulky components with challenging weight distributions in an environment where clearances can reach as little as 3 inches on either side. Lowering detector components down the Homestake mine’s Ross shaft will take four months.
Two configurations
The two far-detector modules needed for phase one of the DUNE experiment will use the same LArTPC technology, though with different anode and high-voltage configurations. A “horizontal-drift” far detector will use 150 6 m-by-2.3 m anode plane assemblies (APAs). Each will be wound with 4000 150 μm diameter copper-beryllium wires to collect ionisation signals from neutrino interactions with the argon.
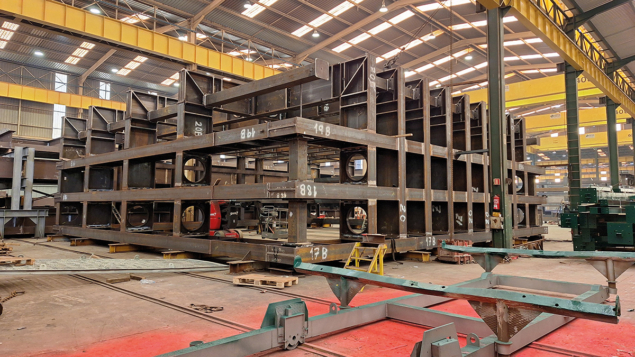
A second “vertical-drift” far detector will instead use charge readout planes (CRPs) – printed circuit boards perforated with an array of holes to capture the ionisation signals. Here, a horizontal cathode plane will divide the detector into two vertically stacked volumes. This design yields a slightly larger instrumented volume, which is highly modular in design, and simpler and more cost-effective to construct and install. A small amount of xenon doping will significantly enhance photo detection, allowing more light to be collected beyond a drift length of 4 m.
The construction of the horizontal-drift APAs is well underway at STFC Daresbury Laboratory in the UK and at the University of Chicago in the US. Each APA takes several weeks to produce, motivating the parallelisation of production across five machines in Daresbury and one in Chicago. Each machine automates the winding of 24 km of wire onto each APA (see “Wind it up” image). Technicians then solder thousands of joints and use a laser system to ensure the wires are all wound to the required tension.
Two large ProtoDUNE detectors at CERN are an essential part of developing and validating DUNE’s detector design. Four APAs are currently installed in a horizontal-drift prototype that will take data this summer as a final validation of the design of the full detector. A vertical-drift prototype (see “Vertical drift” image) will then validate the production of CRP anodes and optimise their electronics. A full-scale test of vertical-drift-detector installation will take place at CERN later this year.
Phase transition
Alongside the deployment of two additional far-detector modules, phase two of the DUNE experiment will include an increase in beam power beyond 2 MW and the deployment of a more capable near detector (MCND) featuring a magnetised high-pressure gaseous-argon TPC. These enhancements pursue increased statistics, lower energy thresholds, better energy resolution and lower intrinsic backgrounds. They are key to DUNE’s measurement of the parameters governing long-baseline neutrino oscillations, and will expand the experiment’s physics scope, including searches for anomalous tau-neutrino appearance, long-lived particles, low-mass dark matter and solar neutrinos.
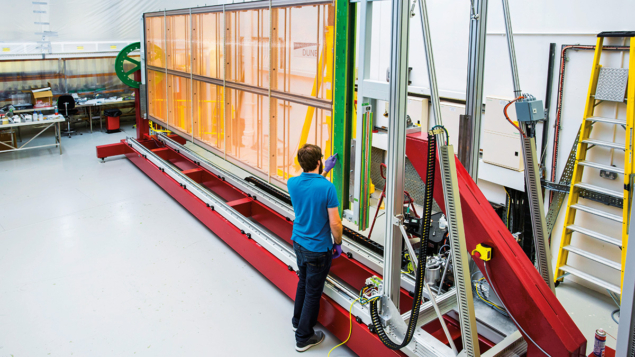
Phase-one vertical-drift technology is the starting point for phase-two far-detector R&D – a global programme under ECFA in Europe and CPAD in the US that seeks to reduce costs and improve performance. Charge-readout R&D includes improving charge-readout strips, 3D pixel readout and 3D readout using high-performance fast cameras. Light-readout R&D seeks to maximise light coverage by integrating bare silicon photomultipliers and photoconductors into the detector’s field-cage structure.
A water-based liquid scintillator module capable of separately measuring scintillation and Cherenkov light is currently being explored as a possible alternative technology for the fourth “module of opportunity”. This would require modifications to the near detector to include corresponding non-argon targets.
Intense work
At Fermilab, site preparation work is already underway for LBNF, and construction will begin in 2025. The project will produce the world’s most intense beam of neutrinos. Its wide-band beam will cover more than one oscillation period, allowing unique access to the shape of the oscillation pattern in a long-baseline accelerator-neutrino experiment.
LBNF will need modest upgrades to the beamline to handle the 2 MW beam power from the upgrade to the Fermilab accelerator complex, which was recently endorsed by P5. The bigger challenge to the facility will be the proton-target upgrades needed for operation at this beam power. R&D is now taking place at Fermilab and at the Rutherford Appleton Laboratory in the UK, where DUNE’s phase-one 1.2 MW target is being designed and built.
The next generation of big neutrino experiments promises to bring new insights into the nature of our universe
DUNE highlights the international and collaborative nature of modern particle physics, with the collaboration boasting more than 1400 scientists and engineers from 209 institutions in 37 countries. A milestone was achieved late last year when the international community came together to sign the first major multi-institutional memorandum of understanding with the US Department of Energy, affirming commitments to the construction of detector components for DUNE and pushing the project to its next stage. US contributions are expected to cover roughly half of what is needed for the far detectors and the MCND, with the international community contributing the other half, including the cryostat for the third far detector.
DUNE is now accelerating into its construction phase. Data taking is due to start towards the end of this decade, with the goal of having the first far-detector module operational before the end of 2028.
The next generation of big neutrino experiments promises to bring new insights into the nature of our universe – whether it is another step towards understanding the preponderance of matter, the nature of the supernovae explosions that produced the stardust of which we are all made, or even possible signatures of dark matter… or something wholly unexpected!
Further reading
DUNE Collab. 2022 JINST 17 P01005.