Colleagues and friends recall the work of the Nobel laureate.

Simon van der Meer was born in 1925 in The Hague, the third child of Pieter van der Meer and Jetske Groeneveld. His father was a school teacher and his mother came from a teacher’s family. Good education was highly prized in the van der Meer family and the parents made a big effort to provide this to Simon and his three sisters. Having attended the gymnasium (science section) in The Hague, he passed his final examination in 1943 – during German occupation in wartime. He stayed at the gymnasium for another two years because the Dutch universities were closed, attending classes in the humanities section. During this period – inspired by his excellent physics teacher – he became interested in electronics and filled his parents’ house with electronic gadgets.
In 1945 Simon began studying technical physics at Delft University, where he specialized in feedback circuits and measurement techniques. In a way, this foreshadowed his main invention, stochastic cooling, which is a combination of measurement (of the position of the particles) and feedback. The “amateur approach” – to use his own words – that he practiced during his stay at Delft University later crystallized in an ability to see complicated things in a simple and clear manner. In 1952 he joined the highly reputed Philips research laboratory in Eindhoven, where he became involved in development work on high-voltage equipment and electronics for electron microscopes. Then, in 1956, he decided to move to the recently founded CERN laboratory.

As one of his first tasks at CERN, Simon became involved in the design of the pole-face windings and multipole correction lenses for the 26 GeV Proton Synchrotron (PS), which is still in operation today, as the heart of CERN’s accelerator complex. Supervised by and in collaboration with John Adams and Colin Ramm, he developed – in parallel to his technical work on power supplies for these big magnets – a growing interest in particle physics. He worked for a year on a separated antiproton beam, an activity that triggered the idea of the magnetic horn – a pulsed focusing device for charged particles, which traverse a thin metal wall in which a pulsed high current flows. Such a device is often referred to as a “current sheet lens”. The original application of the magnetic horn was for neutrino physics. Of the secondary particles emerging from a target hit by a high-energy proton beam, the horn selectively focused the pions. When the pions then decayed into muons and neutrinos, an equally focused and intense neutrino beam was obtained. The magnetic horn found many applications all around the world, for both neutrino physics and the production of antiprotons.
In 1965 Simon joined the group led by Francis Farley working on a g-2 experiment for the precision measurement of the magnetic moment of the muon. There, he took part in the design of a small storage ring (the g-2 ring) and participated in all phases of the experiment. As he stated later, this period was an invaluable experience not only for his scientific life but also through sharing the vibrant atmosphere at CERN at the time – which was full of excitement – and the lifestyle of experimental high-energy physics. It was also about this time, in 1966, that Simon met his future wife, Catharina Koopman, during a skiing excursion in the Swiss mountains. In what Simon later described as “one of the best decisions of my life”, they married shortly afterwards and had two children, Ester (born 1968) and Mathijs (born 1970).
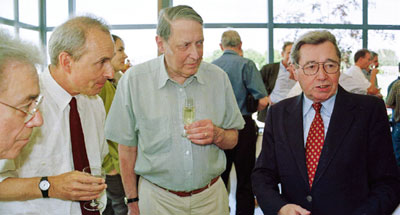
In 1967, Simon again became responsible for magnet power supplies, this time for the Intersecting Storage Rings (ISR) and a little later also for the 400 GeV Super Proton Synchrotron (SPS). During his activities at the ISR he developed the now famous “van der Meer scan”, a method to measure and optimize the luminosity of colliding beams. The ISR was a collider with a huge intensity, more than 50 A direct current of protons per beam, and it was in 1968 – probably during one of the long nights devoted to machine development – that a new and brilliant idea to increase luminosity was conceived: the concept of stochastic cooling.
A Nobel concept
“The cooling of a single particle circulating in a ring is particularly simple” (van der Meer 1984), provided that it can be seen in all of the electronic noise from the pick-up and the preamplifiers. “All” that is needed is to measure the amount of betatron oscillation at a suitable location in the ring and correct it later with a kicker at a phase advance of an odd multiple of 90° (figure 1). But the devil (closely related to Maxwell’s demon) is in the detail. Normally, it is not possible to measure the position of just one particle because there are so many particles in the ring that a single one is impossible to resolve. So, groups of particles – often referred to as beam “slices” or “samples” – must be considered instead.

For such a beam slice, it is indeed possible to measure the average position with sufficient precision during its passage through a pick-up and to correct for this when the same slice goes through a kicker. However, the particles in such a slice are not fixed in their relative position. Because there is always a spread around the central momentum, some particles are faster and others are slower. This leads to an exchange of particles between adjacent beam slices. This “mixing” is vital for stochastic cooling – without it, the cooling action would be over in a few turns. Stochastic cooling does eventually act on individual particles. With the combination of many thousands of observations (many thousands of turns), a sufficiently large bandwidth of the cooling system’s low-noise (sometimes cryogenic) electronics and powerful kickers, it works.
At the time, there were discussions about a possible clash with Liouville’s theorem, which states that a continuum of charged particles guided by electromagnetic fields behaves like an incompressible liquid. In reality, particle beams consist of a mixture of occupied and non-occupied phase space – much like foam in a glass of beer. Stochastic cooling is not trying to compress this “liquid” but rather it separates occupied and non-occupied phase space, in a way similar to foam that is settling. Once these theoretical questions were clarified there were still many open issues, such as the influence of noise and the required bandwidth. With a mild push from friends and colleagues, Simon finally published the first internal note on stochastic cooling in 1972 (van der Meer 1972).
ICE was a storage ring built from components of the g-2 experiment
Over the following years, the newly born bird quickly learnt to fly. A first proof-of-principle experiment was carried out in the ISR with a quickly installed stochastic cooling system. Careful emittance measurements over a long period showed the hoped-for effect (Hübner et al. 1975). Together with the proposal to stack antiprotons for physics in the ISR (Strolin et al. 1976) and for the SPS-collider (Rubbia et al. 1977), this led to the construction of the Initial Cooling Experiment (ICE) in 1977. ICE was a storage ring built from components of the g-2 experiment. It was constructed expressly for a full-scale demonstration of stochastic cooling of beam size and momentum spread (electron-cooling was tried later on). In addition, Simon produced evidence that “stochastic stacking” (stacking in momentum space with the aid of stochastic cooling) works well as a vital tool for the production of large stacks of antiprotons (van der Meer 1978).

Once the validity of the method had been demonstrated, Simon’s idea rode on the crest of a wave of large projects that took life at CERN. There was the proposal by David Cline, Peter McIntyre, Fred Mills and Carlo Rubbia to convert the SPS into a proton–antiproton collider. The aim was to provide experimental evidence for the W and Z particles, which would emerge in head-on collisions between sufficiently dense proton and antiproton bunches. Construction of the Antiproton Accumulator (AA) was authorized and started in 1978 under the joint leadership of Simon van der Meer and Roy Billinge. The world’s first antiproton accumulator started up on 3 July 1980, with the first beam circulating the very same evening, and by 22 August 1981 a stack of about 1011 particles had been achieved (Chohan 2004). The UA1 and UA2 experiments at the SPS had already reported the first collisions between high-energy proton and antiproton bunches in the SPS, operating as a collider, on 9 July 1981.
The real highlight arrived in 1982 with the first signs of the W boson, announced on 19 January 1983, to be followed by the discovery of the Z’ announced in May. This was swiftly followed by the award of the Nobel Prize in physics in 1984 to Simon and Carlo Rubbia for “their decisive contributions to the large project which led to the discovery of the field particles W and Z, communicators of the weak force.”
Simon participated actively in both the commissioning and the operation of the AA and later the Antiproton Accumulator Complex (AAC) – the AA supplemented by a second ring, the Antiproton Collector (AC). He contributed not only to stochastic cooling but to all aspects, for example writing numerous, highly appreciated application programs for the operation of the machines.
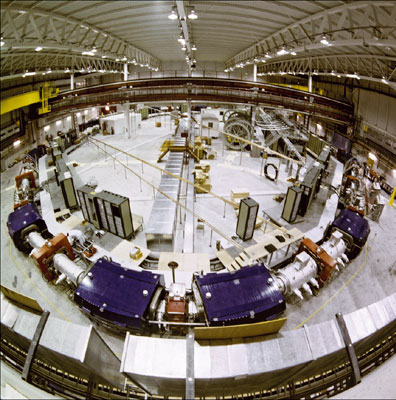
He was certainly aware of his superior intellect but he took it as a natural gift, and if someone else did good work, he valued that just as much. When there was a need, he also did “low level” work. Those who worked with him remember many occasions when someone had a good suggestion on how to improve a controls program, Simon would say, “Yes, that would be better indeed”, and next morning it was in operation. He was often in a thoughtful mode, contemplating new ideas and concepts. Usually he did not pass them on to colleagues for comments until he was really convinced himself that they would work. Once he was sure that a certain concept was good and that it was the right way to go, he could be insistent on getting it going. He rarely made comments in meetings, but when he did say something it carried important weight. He was already highly respected long before he became famous in 1984.
Cooling around the world
In the following years, Simon was extremely active in the conversion and the operation of the AA, together with the additional large-acceptance collector ring, the AC. These two rings, with a total of 16 stochastic cooling systems, began antiproton production in 1987 as the AAC – and remained CERN’S work-horse for antiproton production until 1996. Later, the AA was removed and the AC converted into the Antiproton Decelerator (AD), which has run since 2000 with just three stochastic-cooling systems. These remaining three systems operate at 3.5 GeV/c and 2 GeV/c respectively during the deceleration process and are followed by electron cooling at lower momentum.
Stochastic cooling was also used in CERN’s Low Energy Antiproton Ring (LEAR) in combination with electron cooling until the mid-1990s. In a nutshell, stochastic cooling is most suited to rendering hot beams warm and electron cooling makes warm beams cold. Thus the two techniques are, in a way, complementary. As a spin-off from his work on stochastic cooling, Simon proposed a new (noise assisted) slow-extraction method called “stochastic extraction”. This was first used at LEAR, where it eventually made possible spills of up to 24-hour duration. Prior to that, low-ripple spills could last at best a few seconds.

Simon would see the worldwide success of his great inventions not only before his retirement in 1991, but also afterwards. Stochastic cooling systems became operational at Fermilab around 1980 and later, in the early 1990s, at GSI Darmstadt and For-schungszentrum Jülich (FZJ), as well as at other cooling rings all over the world. The Fermilab antiproton source for the Tevatron started operation in 1985. It is in several aspects similar to the CERN AA /AC, which it has since surpassed in performance, leading to important discoveries, including that of the top quark.
For many years, routine application of stochastic cooling was limited to coasting beams, and stochastic cooling of bunched beams in large machines remained a dream for more than a decade. However, having mastered delicate problems related to the saturation of front-end amplifiers and subsequent intermodulation, bunched stochastic cooling is now in routine operation at Fermilab and at the Relativistic Heavy Ion Collider at Brookhaven. Related beam-cooling methods, such as optical stochastic cooling, are also being proposed or under development.
The magnetic horn, meanwhile, has found numerous applications in different accelerators. The van der Meer scan is a vital tool used for LHC operation and stochastic extraction is used in various machines, for example in COSY at FZJ (since 1996).
After his retirement, Simon kept in close contact with a small group of his former colleagues and friends and there were more or less regular “Tuesday lunch meetings”.
“Unlike many of his Nobel colleagues, who almost invariably are propelled to great achievements by their self confidence, van der Meer remained a modest and quiet person preferring, now that he had retired, to leave the lecture tours to other more extrovert personalities and instead look after his garden and occasionally see a few friends. Never has anyone been changed less by success”, wrote Andy Sessler and Ted Wilson in their book Engines of Discovery (Sessler and Wilson, 2007). At CERN today, Simon’s contributions continue to play a significant role in many projects, from the LHC and the CERN Neutrinos to Gran Sasso facility to the antimatter programme at the AD – where results last year were honoured with the distinction of “breakthrough of the year” by Physics World magazine.
We all learnt with great sadness that Simon passed away on 4 March 2011. He will stay alive in our memories for ever.