The completion of the accelerator technical design report for the proposed Circular Electron–Positron Collider in China marks a milestone towards construction, write Jie Gao, Yuhui Li and Chenghui Yu.
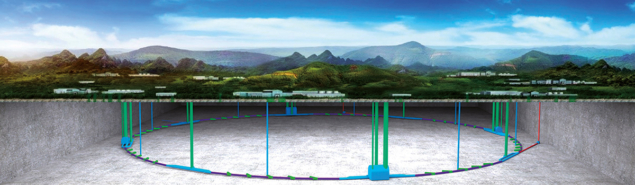
To uncover the fundamental laws of the universe and its evolution is a great human endeavour. The most effective way to achieve this goal in particle physics is via powerful, high-energy accelerators. The July 2012 discovery at CERN of the Higgs boson with a mass of 125 GeV opened a door to an unknown part of the universe. The Higgs boson is not only at the heart of the Standard Model (SM) but is also at the centre of many mysteries. These include the large hierarchy between the weak and the Planck scales, the nature of the electroweak phase transition, the origin of mass, the naturalness problem, the stability of the vacuum, and many other related fundamental questions about nature beyond the SM, such as the origin of the matter–antimatter asymmetry and the nature of dark matter.
Precise measurements of the Higgs boson’s properties serve as probes into the underlying fundamental physics principles of the SM and beyond. For this reason, in September 2012 Chinese scientists proposed the Circular Electron–Positron Collider (CEPC) and the Super proton–proton Collider (SppC) as an international, large science project hosted in China to match the grand goals of particle physics, complementary to linear and muon colliders. Around the same time, physicists at CERN proposed the Future Circular Collider (FCC) staged across electron-positron (e+e–) and hadron-hadron operations.
Since then, the global high-energy physics community has reached consensus on the importance of an e+e– Higgs factory as the next collider after the LHC. In Europe, the 2020 update of the European strategy for particle physics concluded that a Higgs factory is the highest priority, while the US Snowmass 2021 community study and subsequent P5 report released in December 2023 also stressed the importance of overseas Higgs factories. CEPC scientists have actively contributed to both exercises. Meanwhile in Japan, which proposed to host an International Linear Collider (ILC) Higgs factory in 2012, a new baseline design to start at a collision energy of 250 GeV instead of 500 GeV was presented in 2017.
In China, both the 464th and 572th Xiangshan Science Conferences in 2013 and 2016 concluded that “CEPC is the best approach and a major historical opportunity for the national development of an accelerator-based high-energy physics programme”. In 2023, CEPC was identified as the top future particle accelerator in the planning study conducted by the Chinese Academy of Sciences (CAS). This followed an April 2022 statement by the International Committee for Future Accelerators (ICFA) that “reconfirms the international consensus on the importance of a Higgs Factory as the highest priority for realising the scientific goals of particle physics” and expressed support for Higgs-factory proposals worldwide. Five years after the publication of a conceptual design report in November 2018, a technical design report (TDR) for the CEPC accelerator – numbering more than 1000 pages and representing the first such report for a Higgs factory based on a circular collider – has now been completed.
CEPC is a circular Higgs factory comprising four accelerators: a 30 GeV linac, a 1.1 GeV damping ring, a booster with an energy up to 180 GeV, and a collider operating at four different energy modes corresponding to ZH production (240 GeV), the Z-pole (91 GeV), the W+W– threshold (160 GeV) and the tt threshold (360 GeV). The machines are connected by 10 transport lines. While the linac and damping ring would be constructed on the surface, the booster and collider would be situated in an underground ring with a circumference of 100 km, reserving space for a later hadron collider, SppC.
CEPC in focus
The CEPC collider features a double-ring structure, with electron and positron beams circulating in opposite directions in separate beam pipes and colliding at two interaction points where large detectors will be installed. The 100 km-circumference full-energy CEPC booster, positioned atop the collider in the same tunnel, functions as a synchrotron featuring a 30 GeV injection energy and an extraction energy equal to the beam collision energy. To maintain constant luminosity, top-up injection will be employed. The 1.8 km-long linac, which serves as an injector to the booster, accelerates both electrons and positrons using S- and C-band radio-frequency systems, equipped with a damping ring to reduce positron emittance. As an alternative option, polarisation schemes are also under study.
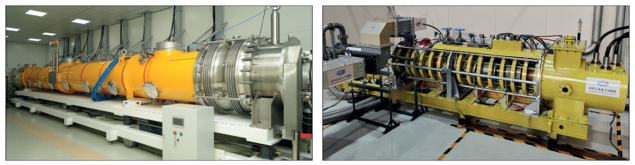
A follow-up to CEPC is SppC, a proton–proton collider with a centre-of-mass energy of up to 125 TeV. The tunnel, primarily consisting of hard rock that will be excavated using either a tunnel boring machine or drill-and-blast methods, allows the SppC to be installed without removing the CEPC. This unique layout opens exciting long-term possibilities for electron–proton and electron–ion physics in addition to the CEPC’s e+e– and the SppC’s proton–proton and ion–ion physics operations. Furthermore, the CEPC would be configured to operate as a high-energy synchrotron-radiation light source with two gamma-ray beamlines, extending the usable synchrotron-radiation spectrum to unprecedented energy (from 100 keV to more than 100 MeV) and brightness ranges. The 30 GeV injection linac can also produce a high-energy X-ray free electron laser by adding an undulator.
Dedicated goals
The CEPC operation plan and physics goals follow a “10-2-1-5” scheme, dedicating 10 years as a Higgs factory, two years as a Z factory, one year as a W factory and possibly an additional five years’ operation at the tt threshold. The four collision modes (corresponding to H, Z, WW and tt production) have a baseline synchrotron radiation power of 30 MW per beam. Luminosity upgrades are also considered by increasing the synchrotron-radiation power per beam up to 50 MW, reaching a luminosity of 8 × 1034 at 240 GeV. With the upgraded luminosity plan, 4.3 million Higgs bosons, 4.1 trillion Z bosons, 210 million W bosons and 0.6 million tt pairs would be produced in the two CEPC detectors.
After the completion of the CEPC conceptual design report, the accelerator entered a five-year-long TDR study during which the design was further optimised. The resulting report, released on 25 December 2023, emphasises the optimal luminosity, coverage of H, Z, W and tt energies, and the full spectrum of technology R&D, civil-engineering designs, industrial and international collaborations and participation.
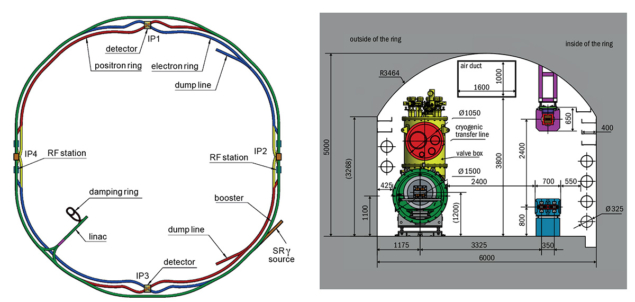
Smaller emittances at the interaction points have been adopted to increase the luminosities, dynamic apertures including various errors for four energies match the design goals, beam–beam and collective effects have been verified, and the machine–detector interface has been optimized with a 20 cm-diameter central beryllium pipe at the interaction points. The booster has adopted a theoretical minimum emittance-like lattice design with an injection energy raised to 30 GeV and output energy of up to 180 GeV.
CEPC accelerator R&D has been conducted in synergy with the fourth-generation 6 GeV High Energy Photon Source project at IHEP in Beijing. These R&D activities cover the collider and booster magnets, superconducting quadrupoles for the insertion regions, NEG-coated vacuum chambers, superconducting cryomodules, cryogenic systems, continuous-wavelength high-efficiency klystrons, magnet power supplies, mechanics, S-band and C-band linac and positron source, damping ring, instrumentation and feedbacks, control system, survey and alignment, radiation protection and environmental aspects.
CEPC is the best approach and a major historical opportunity for the national development of an accelerator-based high-energy physics programme
As three examples, firstly the CEPC booster 1.3 GHz 8 × 9-cell cavity cryomodule has been shown to reach a quality factor/accelerating gradient of 3.4 × 1010/23 MVm–1, surpassing the booster specification (see “CEPC technologies”, left image). Secondly, the CEPC 650 MHz one-cell cavity reached 2.3 × 1010/41.6 MVm–1 at 2 K with electrical-polishing treatment and 6.3 × 1010/31 MVm–1 with medium-temperature treatment. Thirdly, three collider-ring 650 MHz, 800 kW continuous-wavelength high-efficiency klystrons have been developed at IHEP, where the second klystron (see “CEPC technologies”, right image) has reached an efficiency of 77.2% at 849 kW in pulsed mode compared with the design value of 77% at 800 kW in CW mode. The third klystron is a multibeam klystron with a design goal of 80.5%, and its electron source is currently undergoing tests. The achievements of the CEPC accelerator TDR are also a result of strong industrial participation and contributions, via the CEPC industrial promotion consortium.
High-energy ambitions
As part of future strategic technology R&D in high-energy physics and beyond, the CEPC team has proposed an alternative beam-driven plasma injector with beam energies from 10 to 30 GeV. To develop and demonstrate the necessary plasma technologies, such as positron acceleration, staged acceleration and high beam qualities for future linear colliders, IHEP initiated a plasma acceleration experimental programme in September 2023 using the injector linac of BEPCII (a 1.89 GeV e+e– collider with a luminosity of 1033 cm–2s–1) and experimental facilities funded by CAS to the tune of RMB 0.12 billion ($17 million).
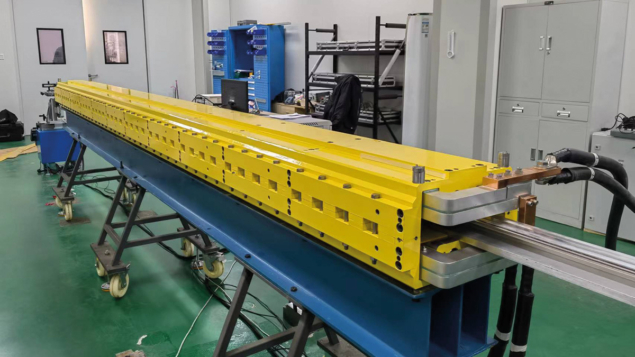
The SppC, in conjunction with the CEPC, would not only provide unprecedented precision on Higgs-boson measurements but explore a significantly larger region of the new-physics landscape, propelling our understanding of the physical world to new heights. A future hadron collider is both more costly than a Higgs factory and more technically challenging. Critical issues such as high-field (20 T or higher) superconducting magnets, synchrotron radiation in a cryogenic environment and a sophisticated beam-collimation system for quench protection must be adequately addressed before construction can begin.
High-field magnets based on high-temperature iron-based superconductors are proposed as the key development path for the SppC. This technology has a much higher magnetic field potential (>30 T) and lower cost than the NbTi/Nb3Sn technologies used nowadays, and significant progress has been made, together with industry, during the past eight years. In 2016 more than 100 m of iron-based “7-core” tape was fabricated, reaching a current density of 450 A/mm2 at 10 T and 4.2 K in 2022.
The SppC is expected to achieve a peak luminosity of 1035 cm–2s–1 per interaction point and an integrated luminosity of approximately 30 ab–1, assuming two interaction points and a runtime of 20–30 years. To further reduce the energy consumption of SppC and CEPC (which has a total power consumption of 262 MW at the ZH energy with a synchrotron-radiation power of 30 MW per beam), various countermeasures are under study.
From 2019 to 2022, CEPC accelerator activities were guided by an International Accelerator Review Committee. In June and September 2023, the CEPC accelerator international TDR and cost review were carried out at Hong Kong University of Science and Technology, while the civil-engineering cost was reviewed by a domestic committee in June 2023. The total CEPC cost is estimated at RMB 36.4 billion ($5.15 billion), with accelerator, infrastructure and experiments taking up RMB 19 billion, 10.1 billion and 4 billion, respectively. Among all the CEPC candidate sites, three – Qinhuangdao, Huzhou and Changsha – have been studied in the TDR.
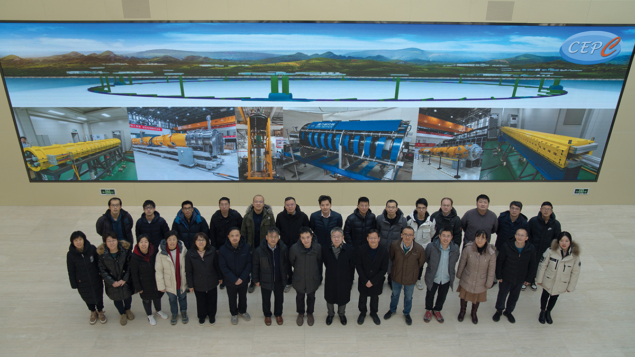
At the end of October 2023, the CEPC international advisory committee supported the conclusion of the TDR review that the accelerator team is well prepared to enter an engineering design report (EDR) phase. The following month, CEPC–SppC proposals were presented at the ICFA Seminar at DESY, declaring the completion of the CEPC accelerator TDR.
Concerning the technology and status of the CEPC detectors, a full spectrum R&D programme has been carried out, spanning the pixel vertex detector, silicon tracker, time projection chamber and drift chamber, time-of-flight detector, calorimeters, high-temperature superconducting solenoid and mechanical design, among others. This R&D also benefits from past experiences with BESIII (in particular concerning the drift chamber and superconducting magnet) and from the High-Luminosity LHC upgrades for ATLAS and CMS (such as the silicon-strip detector and high-granularity calorimeter). The CEPC detector TDR reference design began in January 2024 and will be completed in mid-2025 within the EDR phase (2024–2027).
EDR and schedule
The aim is to present the CEPC proposal (including accelerator, detector and engineering) for selection by the Chinese government around 2025, with construction to start in around 2027 and to be completed around 2035. A preliminary accelerator EDR plan has been established and is to be reviewed by the International Accelerator Review Committee in 2024.
The SppC, in conjunction with the CEPC, would propel our understanding of the physical world to new heights
Concerning CEPC development towards construction, CAS is planning for China’s 15th “five-year plan” for large science projects, for which a steering committee chaired by the CAS president was established in 2022. High-energy physics and nuclear physics, one of eight fields in the plan, has selected nine proposals that have been reviewed in an open and international way. CEPC is ranked first, with the smallest uncertainties by every committee (including domestic committees and an international advisory committee). A final report has been submitted to CAS for consideration.
CEPC has always been envisioned as an international big-science project, and participation is warmly welcomed both in scientific and industrial ways. The CEPC accelerator TDR represents the efforts of thousands of domestic and overseas scientists and engineers. Such a facility would play an important role in future plans of the worldwide high-energy physics community, deepening our understanding of matter, energy and the universe to an unprecedented degree while facilitating extensive research and collaboration to explore the frontiersof technology.
Further reading
CEPC Study Group 2018 arXiv:1809.00285 and 1811.10545.
CEPC Accelerator Study Group 2019 arXiv:1901.03169 and 1901.03170.
CEPC Accelerator Study Group 2022 arXiv:2203.09451 and 2205.08553.
CEPC Study Group 2023 arXiv:2312.14363.