A new laser system that could drive plasma acceleration.
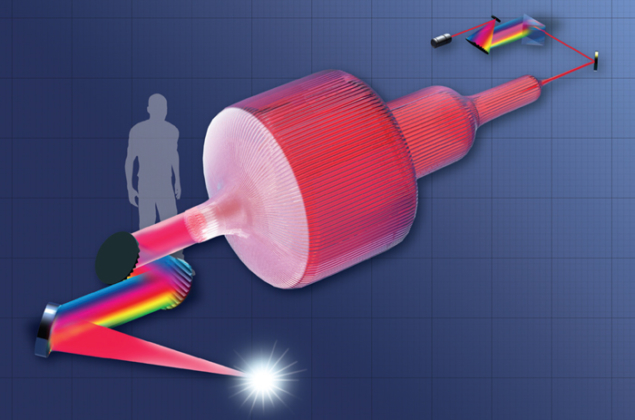
Image credit: Phil Saunders/spacechannel.org.Image credit: Phil Saunders/spacechannel.org.
The interaction with plasma of ultra-high-peak-power lasers at the terawatt to petawatt level has the potential to generate large accelerating gradients of giga-electron-volts per metre. Laser-plasma acceleration could therefore be an important replacement for present technology. However, there are two formidable hurdles to overcome: a laser repetition rate that is limited to a few hertz leading to an average power of only a few watts and a dismal wall-plug efficiency of a fraction of a per cent. This twin technological challenge must be resolved if laser wakefield acceleration is to be considered for large-scale applications in science and society.
On 27–28 June, the International Coherent Amplification Network (ICAN) Consortium concluded its EU-supported 18-month feasibility study with a final symposium at CERN that was organized by Ecole Polytechnique, the University of Southampton, Fraunhofer IOF Jena and CERN. A major topic concerned progress with the novel laser architecture known as coherent amplification network (CAN), which could for the first time provide petawatt peak power at a repetition rate as high as 10 kHz. This corresponds to an average power in the megawatt range with an efficiency better than 30% and opens the door to many applications.
The ICFA-ICUIL Joint Task Force (JTF) produced a report noting that while the science of laser acceleration has matured, the technology for intense lasers is lagging behind
The symposium also looked at the path towards future laser-driven accelerators and colliders, applications in free-electron lasers and neutron/neutrino sources, as well as the laser search for the “dark fields” of dark matter and dark energy. Other topics included compact proton accelerators that could be used for accelerator-driven systems for nuclear energy or for hadron therapy, as well as the generation of γ-ray beams with a host of applications, from the identification of isotopes in exposed spent nuclear fuel – such as at Fukushima – to nuclear pharmacology.
The main paradigm currently driving fundamental high-energy physics is the high-energy charged-particle collider. To apply laser-acceleration methods to a future collider, the international communities involved in intense lasers and high-energy physics – in the form of the International Committee on Ultra-High Intensity Lasers (ICUIL) and the International Committee for Future Accelerators (ICFA), respectively – came together in 2009 to form a collaborative working group to study how best to proceed. The ICFA-ICUIL Joint Task Force (JTF) produced a report noting that while the science of laser acceleration has matured, the technology for intense lasers is lagging behind, specifically in the development of systems with a high repetition rate and high efficiency (Leemans et al. 2011).
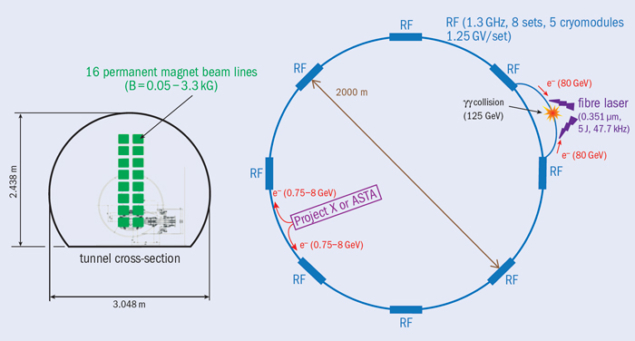
Image credit: Chou et al. 2013.
The possibility of amplifying laser pulses to extreme energy and peak power not only offers a route to a more compact and cheaper way to perform high-energy physics, it could also open the door to a complementary research area that is underpinned by single-shot, high-field laser pulses – a new field of (laser-based) high-field fundamental physics (Tajima and Dawson 1979 and Mourou et al. 2006). To muster the support of the scientific community for this vision, the International Center on Zetta-Exawatt Science and Technology (IZEST) was set up in 2011 and now has 23 associated institutes worldwide.
The ICAN project
Stimulated by the JTF’s report, members of IZEST set up the ICAN project, which involves a total of 16 institutes including Ecole Polytechnique, the University of Southampton, Fraunhofer IOF Jena and CERN as beneficiaries with a further 13 institutes participating as experts. Starting in October 2011, ICAN began research on the development of the fibre-laser-based CAN concept. Here, pulses from thousands of fibre lasers – built on technology that was originally developed for the telecommunications industry and each capable of producing low-energy pulses efficiently and at a high repetition rate – are coherently added to increase the average power and pulse energy linearly as the number of fibres increases (figure 1). The ICAN project has shown that this architecture can provide a plausible path towards the necessary conditions for a high-energy collider based on a laser accelerator, so answering the challenge that was posed by the JTF report. The fibre laser offers excellent efficiency (>30%) thanks to laser-diode pumping and provides a much larger surface cooling area, therefore making operation at high average power possible.
The most stringent requirement is to phase all of the lasers within a fraction of a wavelength. This originally seemed insurmountable but a preliminary proof of concept that was discussed earlier this year in Nature Photonics suggests that tens of thousands of fibres can be controlled to provide a laser output that is powerful enough to accelerate electrons to energies of several giga-electron-volts at a 10 kHz repetition rate. This is an improvement of at least 10,000 times on today’s state-of-the-art (Mourou et al. 2013). Furthermore, experiments have demonstrated the feedback control of the phase and timing of pulses from each fibre to the attosecond precision necessary for coherent addition. This means that the spatial profile of the overall laser pulse can be delicately controlled to provide a precise beam shape – a highly desirable feature for laser accelerators.
Immediately after the publication of the article in Nature Photonics, the group at Fermilab re-launched the concept of a photon (γ–γ) collider for a Higgs factory, using the CAN laser as the source of low-energy photons to generate high-energy γ rays through inverse Compton scattering from two electron beams (figure 2). Such a collider would have a lower beam energy than the equivalent electron–positron collider and less noise (Chou et al. 2013). The required repetition rate is around 50 kHz, within the reach of CAN technology.
Fundamental physics via high fields
While a photon collider would study Higgs physics and other high-energy phenomena, copious coherent photons from the CAN lasers could also provide a new opportunity to look for undetected “dark fields” that are associated with low-energy dark matter (axion-like particles, for example) and dark energy (Tajima and Homma 2012). The use of three distinct parallel lasers with huge numbers of photons could allow sensitive detection of possible dark fields. The basic idea is akin to degenerate four-wave mixing, well known in traditional nonlinear optics but in this case probing the vacuum, whose possible nonlinear constituent is so weak that it has appeared “dark” until now. Since the parallel injection of lasers can make their beat frequency particularly low, the range of detectable masses is extremely low – down to nano-electron-volts – compared with orthodox high-energy physics experiments. The extremely high equivalent luminosity is also unusual. Because of the coherence of the photons, the luminosity of the events is proportional to the triple products of the numbers of photons from the three lasers – N1N2N3. If the laser has 10 kJ energy, the Ni here can each be as large as 1023. On the other hand, the luminosity of a charged-particle collider is proportional to NaNb, where a and b represent the two beams and these Nj are typically around 1010. The two products that determine the luminosity of each “collider” therefore differ by as much as 50 orders of magnitude.
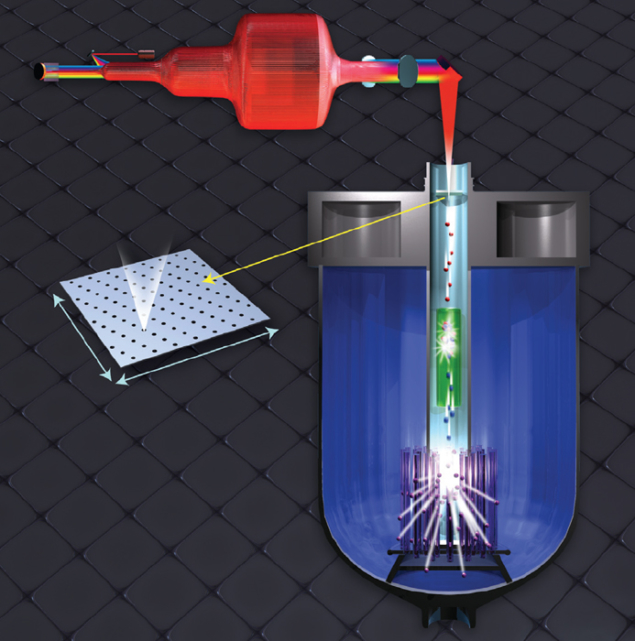
Image credit: Phil Saunders/spacechannel.org.
Laser-driven acceleration processes, laser wakefield acceleration and the related physical processes might also appear in nature, as demonstrated by the recent realization that the accretion disc of a supermassive black hole and its associated jets could be the theatre for extreme high-energy cosmic-ray acceleration up to 1021 eV and accompanying γ-ray emission (Ebisuzaki and Tajima 2013). The disruptive magnetic activities of the disc give rise to the excitation of huge Alfvén waves and the mode-converted electromagnetic waves created in the jet are capable of accelerating ions to extreme energies via a process that is similar to laser acceleration. The coherence of the relativistic waves and particles implies a fundamental similarity between terrestrial and celestial wakefield acceleration processes. It is hoped that one day celestial-type wakefields might be achieved by a similar physical process but on different scales on Earth.
Applications in society
Turning to more earthly issues, the CAN fibre laser opens up many doors to challenging issues in society. With high average power at the same time as high peak power, along with high efficiency and inherent controllability, the CAN source is applicable to many new areas, of which the following are a few examples.
Laser acceleration of protons would provide compact installations compared with conventional accelerators, which could lead to compact proton-therapy accelerators (Habs et al. 2011). As the intensity of the laser becomes highly relativistic and the dynamics of proton acceleration become more relativistic, the acceleration mechanism becomes more efficient and the beam quality improves (Esirkepov et al. 2004). It then becomes plausible to produce an efficient, compact proton source in the relativistic regime.
Laser-driven compact proton beams could also act as the source of neutrons for accelerator-driven systems and accelerator-driven reactors for nuclear energy (figure 3). The high-fluence CAN laser offers the potential for highly efficient compact neutron sources that would be less expensive than those based on conventional methods (Mourou et al. 2013).
Just as in the case of the photon collider but at lower energies, the CAN laser can produce copious γ rays with specified energy in a well defined direction. Such γ-ray sources are useful for detection in nuclear physics, such as in isotopic determination via nuclear resonance fluorescence. Since a CAN-driven γ-ray source could be compact enough to be portable, it could be used for isotopic diagnosis of exposed spent nuclear fuel – as at Fukushima – without contact or proximity. Other industries – auto, chemical, mechanical, medical, energy, etc – have the need for high-fluence, high-efficiency short-pulse lasers. One example is nuclear pharmacology. Since a CAN source produces γ rays (and so neutrons) of specific energy, it can be used to create specific isotopes of nuclei that are known to be useful for medical purposes (Habs et al. 2011).
The future looks bright for fibre lasers – not only in high-energy physics but in many applications for society.