South Korea’s RAON heavy-ion accelerator facility will open up new opportunities in rare-isotope science when it comes online later in the decade. Joe McEntee reports.

With the worst of the supply-chain disruption from the COVID-19 pandemic receding, work is moving at pace on the preparation and systems commissioning of the Rare isotope Accelerator complex for ONline experiments (better known as RAON), the flagship heavy-ion accelerator facility that forms part of the Rare Isotope Science Project (RISP) within South Korea’s Institute for Basic Science.
RAON is big science writ large. By accessing exotic and as-yet-undiscovered radioisotopes, RAON will address a broad-scope research roadmap when it comes online for initial user experiments in two years’ time. By extension, the laboratory will generate a wealth of data to advance physicists’ fundamental understanding of the nucleus; provide novel insights about the origins of the chemical elements in the universe; and enable experiments to explore physics beyond the Standard Model. Equally significant, RAON will produce research quantities of rare isotopes to underpin diverse applied R&D efforts spanning, for example, the diagnosis and treatment of cancer, safe disposal of spent nuclear fuels, and the lossless storage of electrical energy.
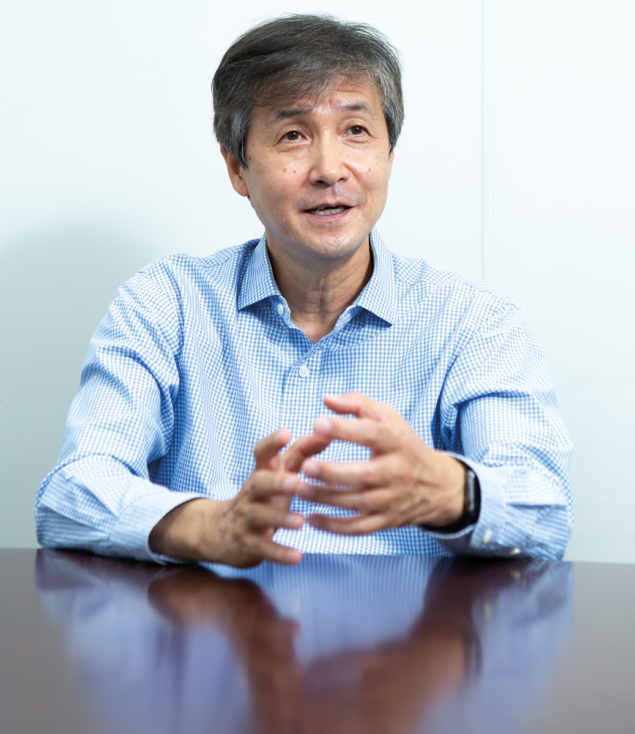
It’s RAON’s combined production scheme, however, that sets it apart from other heavy-ion accelerator laboratories. Specifically, a twin-track approach that exploits – separately as well as in tandem – two methods for producing rare isotopes: isotope separation online (ISOL) and in-flight fragmentation (IF). For context, ISOL involves the acceleration of light ions (e.g. protons) and their collision with a heavy-element target (e.g. uranium), with a large abundance of rare (and high-purity) isotopes extracted following fragmentation of the target. With IF, on the other hand, accelerated heavy ions (e.g. uranium) collide with a light-element target (e.g. carbon), with strong magnets extracting rare isotopes of interest from many kinds of very fast-moving, fragmented heavy-ion beams.
RAON is, by some way, South Korea’s biggest big science endeavour to date
Here Myeun Kwon, director of RISP and RAON, tells CERN Courier how the new facility is taking shape with help from a network of partnerships across the scientific community and industry.
What differentiates RAON’s scientific mission from other heavy-ion accelerator facilities?
We are developing RAON, first and foremost, to access the unexplored regions of the nuclear landscape. Upon completion, RAON will provide a first-of-its-kind production facility, combining ISOL as a first step and IF systems in a second step to produce and study the more exotic radioisotope beams – in fact, up to 80% of all the isotopes predicted to exist for elements below uranium. There are a number of studies – theoretical and experimental – which suggest that such a two-step process will expand the horizon for radioisotope production dramatically.
While other heavy-ion accelerators rely exclusively on either ISOL or IF, RAON will be the first to exploit a combined ISOLIF production scheme – while simultaneously making ISOL and IF available to users as stand-alone processes. As such, RAON is expected to increase the rate of discovery of rare isotopes, whilst producing them in larger quantities and in greater variety.
Building blocks: the RAON accelerator facility
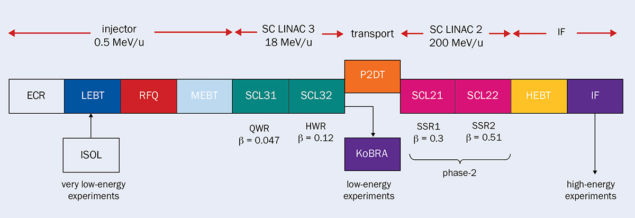
Upon completion, the RAON accelerator will generate heavy and light ion beams at a wide range of momenta – up to 200 MeV/nucleon for uranium and 600 MeV for protons (and with a beam current range from 8.3 pμA for uranium and 660 pμA for protons).
In terms of the core building blocks, RAON comprises an injector system and three discrete superconducting linac sections, the superconducting cavities of which are phased independently and operated at three different frequencies (81.25, 162.5 and 325 MHz).
The low-energy superconducting linac section (SCL3) and the high-energy superconducting linac (SCL2) are connected by a post-accelerator driver linac (P2DT), which consists of a charge-stripper, two rebunchers and a 180° bending system.
The injector system accelerates a heavy-ion beam to 500 keV/nucleon and creates the desired bunch structure for injection into the SCL3 linac.
The injector comprises two electron cyclotron ion sources (ECR-IS), a low-energy beam transport section (LEBT), an RF quadrupole (RFQ) and a medium-energy beam transport system (MEBT).
The LEBT is designed to transport and match ion beams extracted from the ECR-IS to the RFQ; electrostatic quadrupoles are chosen for transport and focusing because these are more suitable for the LEBT’s low-velocity beams.
The RFQ (approx. 5 m long with a four-vane structure) is designed to accelerate ion beams from 10 keV/nucleon to 500 keV/nucleon at 81.25 MHz of the resonance frequency.
The MEBT comprises 11 room-temperature quadrupole magnets to transport and focus the ion beams, with four bunching cavities (operating at 81.25 MHz of resonance frequency) arranged to match the longitudinal beam size to SCL3.
Phase II of the accelerator roll-out (due for completion in 2025) involves the construction and commissioning of the high-energy superconducting linac (SCL2).
Completion of Phase II of RAON will see the launch of a co-located laboratory to evaluate next-generation radiotherapy modalities for the treatment of cancer – for example, the combined use of 11C particle beams for localised radiotherapy and in situ gamma-ray imaging of solid tumours (so-called theranostics).
How important are partnerships – domestic and international – for the successful delivery of the RAON initiative?
RAON is, by some way, South Korea’s biggest big science endeavour to date. Put simply, the project would not be possible without our extensive R&D partnerships, supporting us with the co-development of core enabling technologies for the accelerator facility and the experimental systems for RAON’s front-line research programme. We have a network of Korean universities and research institutes, for example, working on accelerator design and development, as well as the manufacture and testing of superconducting components and subsystems.
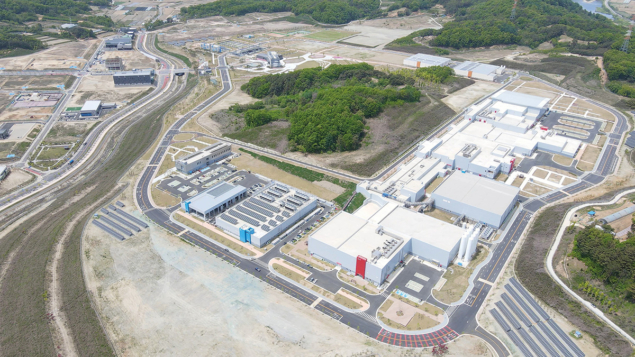
International collaboration is front-and-centre as well, with diverse technology contributions from the likes of TRIUMF (Canada), RIKEN and KEK (both Japan), the Institute of High Energy Physics (IHEP, China), the Institute for Nuclear Physics (INFN, Italy) and the European Spallation Source (ESS, Sweden). At a more strategic level, we rely on broad engagement and oversight from a network of scientific and engineering experts represented on our international/technical supervisory committees.
What does RAON’s engagement with industry look like?
We’re pursuing a mixed model with industry – using off-the-shelf technologies when appropriate to manage our capital outlay, but also co-developing unique breakthrough technologies that can subsequently be transferred and exploited more widely by industry. A good example of the latter is Vitzro Tech, a domestic manufacturer that we engaged on bespoke development and manufacture of a portfolio of niobium superconducting RF cavities, cryomodules and cryogenic distribution lines for key legs of the accelerator facility. Vitzro Tech’s inputs are fundamental to the project’s long-term success and the expectation is that the technologies the company developed for RAON will, in time, be offered commercially to other big science facilities – a case study in downstream innovation.
Presumably, you need to forge close links with equipment manufacturers at home and abroad?
Correct. Big science is all about collaboration, so the priority, from the off, is to have tight communication with your industry vendors. We have domestic manufacturers, for example, that have supplied us with a range of commercially available accelerator technologies – advanced magnets, vacuum systems and associated instrumentation – while international manufacturers also feature prominently in the project supply chain. The RAON cryoplant is a case in a point – a turnkey system developed specifically for RAON by our technology partner Air Liquide of France.
How has the project timeline been affected by the COVID-19 pandemic?
RAON depends on equipment deliveries from regional and international technology partners, so some supply-chain disruption was inevitable as a result of the pandemic. Notwithstanding the logistical obstacles, we have registered significant progress along many coordinates over the past three years. The construction of all buildings and supporting facilities was completed in 2021, while the low-energy linac – which includes two types of superconducting RF cavities – and its associated cryoplant, ISOL facilities (with cyclotron) and experimental systems (seven in all) are also complete.

A significant commissioning milestone was reached in October 2022 with the first argon-ion beams accelerated by the low-energy superconducting linac, with all the linac subsystems – including quarter-wave and half-wave resonator cavities – cooled down to cryogenic temperatures (see “Building blocks: the RAON accelerator facility”). The mechanical installation and commissioning of the associated cryoplant (4.2 kW cooling capacity as the equivalent heat load at 4.5 K) was completed back in August 2022, with the “cold box” connected to the main helium distribution line.
What are the next steps for RAON?
We are now in the middle of commissioning the low-energy superconducting linac and aim to complete that process early next year. We envisage a similar commissioning timeline for the ISOL facility (with 70 MeV proton cyclotron) and the low-energy experimental facilities such as KoBRA (Korea Broad acceptance Recoil Spectrometer and Apparatus). In the middle of 2023, we will combine all of these building blocks for initial radioisotope production, with preparations for the first round of user experiments (at low energies) taking another year or so through to autumn 2024. Phase two of the RAON installation involves the construction and commissioning of the high-energy superconducting linac.