A CERN-inspired development at the Manne Siegbahn Laboratory, Stockholm, has produced physics results of impressive accuracy. Ingmar Bergström reports.

From time to time, people ask whether particle physics at CERN and other large laboratories produces applications for other sectors of society. Usually they are pointed towards the use of detector technology in medicine and the development of information technology. Everyone knows that the World Wide Web was born at CERN, but even earlier than that the very creation of the laboratory was one of the first demonstrations of how effective European co-operation can be.
Less frequently discussed is how CERN has become such a rich source of scientific and technical knowledge from specialists from all over the world. Visitors at CERN exposed to this enormous pool of knowledge and expertise are often inspired with new ideas to implement when they return to their home labs. The impact of large international labs such as CERN is thus boosted by related activities at the smaller home labs.
CERN collaboration case-study
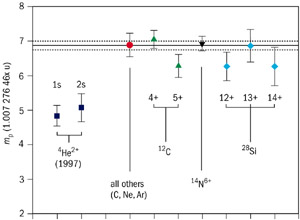
For more than two decades, Stockholm’s Manne Siegbahn Laboratory was one of the collaborators in the study of exotic atoms, first at CERN’s PS and later at LEAR. (In exotic atoms, the orbital electrons of everyday atoms are replaced by other, heavier particles, such as muons, kaons and antiprotons.)
However, we were often motivated to try to transfer some of CERN’s impressive technological achievements to our own home lab, within a necessarily limited budget. Therefore, sabbatical years for scientists and leave of absence for skilled technicians working with CERN accelerator groups, the vacuum group and in the electronics sector were organized systematically.
From this came the idea to build a storage ring where particles did not make head-on collisions as in particle physics but merged in a soft collision. Though stored ions could have energies of tens of mega-electronvolts per nucleon, and, for example, be merged with electrons in the kilo-electronvolt region, the collision energies, surprisingly, could be as small as fractions of a milli-electronvolt.
Stockholm’s CRYRING storage ring was to a great extent based on the design and experience of CERN’s low energy antiproton ring (LEAR), although the size is somewhat smaller (circumference 54 m compared with 80 m for LEAR). Figure 1 shows the CRYRING layout with its ion injectors and low-energy experiments.
An impressive amount of work has been published since CRYRING began running in 1992. From the very beginning, research concentrated on stored highly charged ions produced in an electron-beam ion source (CRYSIS).
CERN asked whether Stockholm could reproduce the seemingly strange LEAR result that the lifetimes of stored lead-208 ions with some even and odd charge states were dramatically different.
CRYRING confirmation
To optimize the beam, the lead ions injected into LEAR were cooled by electrons. This also brings the risk that ions are lost by dielectronic recombination. In such a two-electron resonance process, a cooling electron is captured by the ion and the energy balance is regulated by the excitation of one of the bound electrons. This was studied for 53+ and 54+ charge states by R Schuch and H Danared. Not only were the LEAR results confirmed, but the reason for this deviation was indeed found to be due to dielectron recombination resonances.
In the case of 53+, these occurred at the very low energies of 0.1 and 1 meV. Similar resonances are not present for 54+. From the measurements it is possible to determine the energy splitting between the ground state and the first excited state (4s and 4p levels) with an accuracy of about 1 meV. This is the most accurate determination of such a splitting ever measured in a very highly charged ion. The energy splitting includes large quantum electrodynamic effects.
At the ESR storage ring at the GSI Darmstadt lab, researchers have observed an ordered structure of stored ions when only some 5000 ions are left in the ring – a phenomenon sometimes referred to as one-dimensional ion crystallization. This has also been confirmed at CRYRING by studying the behaviour of stored nickel-17+ ions. Recent work has shown the existence of a one-dimensional lattice of xenon-36+ ions with thousands of stored ions.
In 1985 two experiments using electromagnetic traps were launched at CERN. One, at LEAR (G Gabrielse et al), aimed at a precision determination of the ratio of antiproton to proton masses, and it went on to show that they were equal to within a factor of 9 x 10-11. The other trap experiment (ISOLTRAP) was set up at the ISOLDE on line ion source for the determination of masses of radioactive nuclei. More than 100 masses of short-lived radioactive atoms have been determined to an accuracy of 10-7.
Having an electron beam ion source in Stockholm, we asked why everybody was using singly charged ions when it was obvious that the precision in mass measurements in a Penning trap increases linearly with the ionic charge. In collaboration with H-J Kluge’s group, we built another Penning trap (SMILETRAP) at Mainz, which was subsequently connected to the Stockholm electron beam ion source.
In a Penning trap, the mass of a highly charged ion is determined by measuring its cyclotron frequency and comparing it with the cyclotron frequency of a mass reference ion – often a carbon-12 ion of a suitable charge. The atomic mass is then obtained by correcting for the mass of missing electrons and their electron binding energies.
In addition to carbon ions, we used helium-4, nitrogen-14, neon-20, silicon-28 and argon-40 ions with various high charges as mass references. Their masses are known to an accuracy of about 10-10. Ionized hydrogen molecules were used as carriers for the proton. The ratio between the hydrogen molecule ion and the proton mass is known to better than 0.05 ppb.
Puzzling deviation
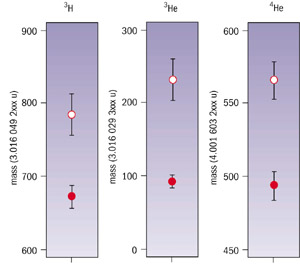
The results are shown in figure 2. The proton mass value obtained (1.007 276 466 72(16)(86)) is close to the currently most accurate value (1.007 276 466 89(13)), except for the mass calculated from helium-4. This deviation seemed puzzling because the listed mass claimed an uncertainty of 0.25 ppb.
We therefore remeasured the helium-3, helium-4 and tritium masses using the hydrogen molecule ion as a mass reference. Figure 3 shows the results. The masses of these three light atoms are heavier than previously reported. It is thought that the relatively large mass discrepancy may be due to a day/night variation in the magnetic field that was unknown at the time of the earlier measurements.
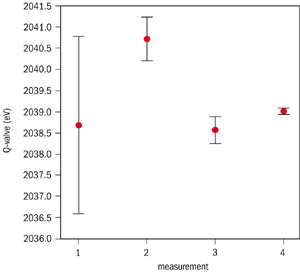
This emphasizes how important it is in precision physics to obtain results from a number of different groups, preferably each employing a different technique. Our interest therefore focused on the neutrinoless double beta decay of germanium-76 – a process that, if observed, would signal some unexpected physics.
The most promising results come from the Heidelberg-Moscow experiment in the Grand Sasso underground laboratory. The masses of germanium-76 and selenium-76 were measured with an uncertainty of 1 ppb. However, for mass difference measurements, the systematic uncertainties cancel and the decay energy equivalent (Q-value) becomes very accurate (Q = 2 039 006(50) eV). Our Q-value is so accurate that its uncertainty represents only a few per cent of the half-width of the expected electron peak in the semiconductor detectors used.
This value may thus seem exaggerated, but it should be pointed out that the mass uncertainty reported from measurements with conventional mass spectrometers often has large underestimated systematic uncertainties. In this particular case, however, we have confirmed the latest reported Q-value (figure 4), and with an accuracy seven times as good.
Through contact with CERN, a number of scientists and technicians at Stockholm have been able to establish a unique facility for advanced in-beam atomic and molecular physics. Other laboratories could produce similar measurements. Our choice of experiments has often been influenced by current problems in particle and accelerator physics – no doubt as a direct result of our contact with CERN.