The best leaders are those who don’t want to be leaders per se, says experimentalist Ian Shipsey, who started out in kaon physics before taking up prominent positions in CLEO, the LHC experiments, the Vera Rubin Observatory and, most recently, quantum technologies.
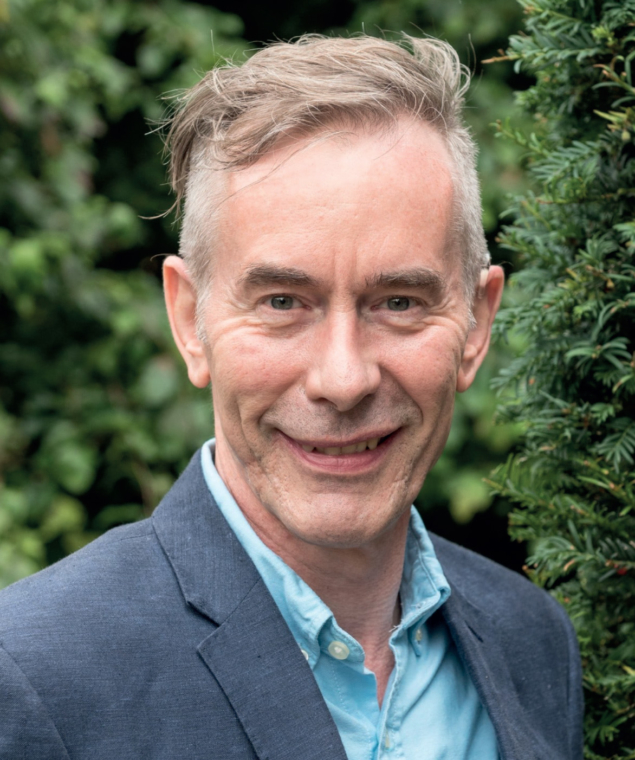
What drew you to particle physics?
Ever since I was an undergraduate, I wanted to know why there was a lot more matter in the universe than antimatter – an asymmetry that permits us to exist. My thesis was on CP violation in the kaon system as part of the NA31 experiment at CERN. I had the opportunity to help build a muon detector and we found the first evidence that matter and antimatter behave differently as they disintegrate; subsequently established with greater significance by NA48 and by KTeV at Fermilab. NA31 was a wonderfully nurturing environment with many brilliant physicists. Like many European students at that time, I was strongly encouraged to head to the US for post-PhD finishing school and decided to join the CLEO experiment at the Cornell Electron Storage Ring (CESR) – for two reasons: first, CLEO studied beauty quarks, which were expected to have much larger CP violating effects than kaons; and second because I had fallen in love with a student (now my wife, Daniela Bortoletto) working on CLEO whom I had met at CERN. CLEO was another astonishingly nurturing environment. I joined Purdue University as an assistant professor just a couple of years after arriving in the US.
How did you make the transition to the LHC experiments?
While I’d help build the CLEO muon spectrometer and worked on analyses, there was an expectation to work on a far-future project as well. I set up a fledgling research group to develop micro pattern gas detectors (MPGDs) for the SDC collaboration at the Superconducting Super Collider (SSC). Fairly quickly we concluded that silicon microstrip and pixel detectors were a better technology choice for this application, but then, in 1993, the SSC was cancelled and a lot of people went towards the LHC. I was invited to join ATLAS due to my MPGD expertise, but I decided to focus on CLEO and the surety of great physics results, which were needed to win tenure. Shortly afterwards, with CLEO colleagues, I received a large grant to build a silicon vertex detector for CLEO III, which was commissioned successfully in 2000. Almost immediately I and my group were invited to join CMS to help build the forward silicon pixel detector. After the pixel detector was installed I was asked to co-lead the LHC Physics Center (LPC) at Fermilab. Then the LHC began operation and I moved to CERN, serving also as the co-convener of the CMS quarkonia working group. The atmosphere at CERN was electric and analysing those first LHC data was one of the most exciting moments of my career. CMS has been a wonderful, supportive environment in which to learn and grow as a physicist. Then, in 2013, I took up a position at Oxford, which is a founding member of ATLAS. I joined ATLAS in 2016 and brought with me experience with muons, silicon and data analysis. It’s very exciting to be part of ATLAS and the collaboration has been very welcoming.
What attracted you to work on the Vera C Rubin Observatory?
The Rubin Observatory is a ground-based 8.4 m, 10 square-degree field-of-view telescope that will see more of the universe at optical wavelengths in its first month of operation than all previous telescopes combined. Scheduled to start in late 2022, (but delayed by COVID-19 situation), it will revolutionise astronomical observations by conducting the Legacy Survey of Space and Time – an optical survey of faint astronomical objects across the entire sky every three nights, enabling precision dark-energy measurements, studies of dark matter and opening a movie-like window on objects that change or move on rapid timescales. I have been a member since 2007, when I was asked to help out in the pitch to the US Department of Energy (DOE) to participate in the project. The scope of particle physics was broadening and the US national laboratories engaged in particle physics had significant capabilities, for example in silicon detector construction, that were an excellent match to the technical challenges of building the Rubin Observatory’s 3 Gigapixel CCD camera. We met healthy scepticism at DOE, given the completely unknown nature of dark energy. From a science perspective we found two lines of argument were useful. First, the job of particle physicists is to understand the fundamental nature of energy, matter, space and time, and in so doing to understand the origin, evolution and fate of the universe. Second, by analogy to the Higgs field and Higgs boson, the cosmological observations are consistent with dark energy being a scalar field, which if correct implies an associated scalar particle. The pitch was successful and the DOE approved funding for the construction of the CCD camera. Soon after arriving at Oxford I was asked to help make the case for UK participation in the project. Like everyone else in the Rubin Observatory community, I am eagerly anticipating first data.
Did you plan to enter scientific management?
I had no plan to be involved in scientific management of any kind! At around the time I joined CMS a few of us had been developing the idea to transform CLEO and CESR into a machine that would preferentially produce charm quarks rather than beauty quarks to test ultra-precise lattice-QCD predictions used by B-physics experiments to extract CKM matrix elements. When getting the idea funded I became the public face of the experiment, and around that time I was also elected by the collaboration to be co-spokesperson.
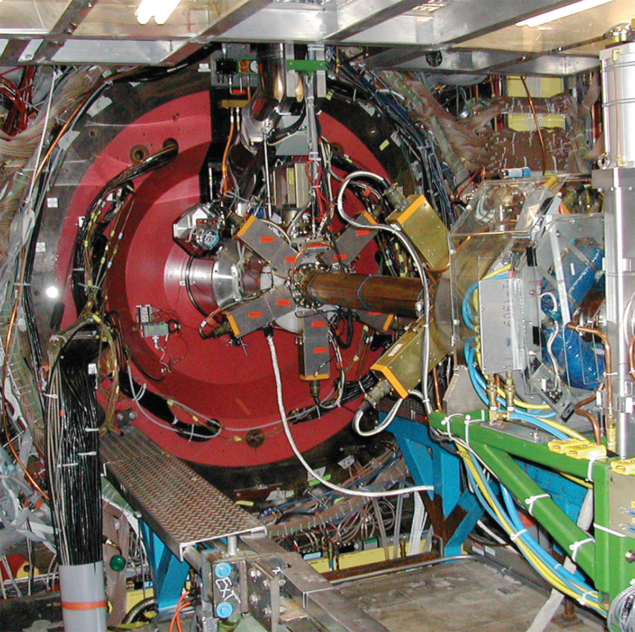
As CLEO entered its twilight phase, the success of the LPC led to me being elected chairperson of the CMS collaboration board in 2012. I was also elected chair of the APS division of particles and fields. Moving back to Europe I was elected head of the Oxford particle-physics group in 2014 and I was elected head of the physics department in 2018. Throughout this entire period, leadership roles have occupied about 50% of my working day, which has meant that to get research done I tend to be connected to my laptop until the early hours on most days. Fortunately, five to six hours of sleep each night is sufficient. I have also been blessed with wonderful colleagues, students, postdocs, and administrative support. In my opinion the best leaders are those people who don’t want to be leaders per se, and I think I was selected for this reason. Particle physics is a team effort, quite distinct to the way an army or a corporation is organised. Our leaders are not generals or CEOs, but colleagues called to serve for a time before returning to the rank and file.
How did you wind up leading the quantum-sensor programme for the UK’s Quantum Technologies initiative?
In 2017 the DOE invited me and a colleague to articulate the case for quantum sensing in particle physics. We co-organised a workshop bringing together many disparate communities from which an influential whitepaper (arXiv.org:1803.11306) emerged and contributed to the creation of a new DOE-funded quantum-sensing programme in 2018. I then conducted a similar activity in the UK at the invitation of the Science and Technology Facilities Council (STFC), bringing together the particle-physics and particle-astrophysics community with the atomic, molecular and optical and condensed-matter communities to form a Quantum Sensing for Fundamental Physics (QSFP) consortium, targeting strategic UK government funding to support interdisciplinary research. STFC announced around £40M for the programme in September 2019 and a call for proposals led to the identification of seven projects for funding, for which an official announcement is imminent. I am a member of one of them: AION (the Atom Interferometer Observatory Network).
What is driving current interest in quantum technologies?
The birth of quantum mechanics nearly 100 years ago has led to the information and communication technology that is now central to modern civilisation – sometimes referred to as the first quantum revolution. But none of the existing technologies use any of the iconic characteristics of quantum mechanics such as the uncertainty principle, superposition states, macroscopic quantum interference, or two-particle quantum entanglement. Second-generation quantum technology that exploits these phenomena is just coming online.
NA31 was a wonderfully nurturing environment with many brilliant physicists
Most well-known is quantum computing, which exhibits extraordinary capabilities and is steadily entering the scientific and corporate marketplaces. As humankind harnesses the characteristics of quantum mechanics and gains mastery over them we will witness the second quantum revolution that will transform our society in as profound a way as the first quantum revolution did. It is no different to the transistor in the 1950s: if people told you back then that transistors could change your life, no one would have believed you; now we have a billion of them in a smart phone. So we can start to harness (crudely) phenomena such as entanglement and the promise is that over the next 20–30 years we can put this technology in your phone. We can’t even begin to think what that would enable because it’s beyond our imagination. Think quantum internet, quantum liquid crystals and quantum artificial neural networks.
What do quantum technologies offer high-energy physics?
A revolution in the theory and tools of quantum mechanics has produced new sensitive measurement techniques that allow measurements to be made near the intrinsic noise limits imposed by the uncertainty principle, as well as enabling new capabilities in sensitivity, resolution and robustness. This can now be harnessed to accelerate searches for new physics including, for example, dark matter, hidden dark sectors and electric dipole moments. For decades, one way that we’ve hunted for dark-matter particles is with large detectors via nuclear recoils, but the allowable mass ranges from 10-22 eV to the Planck scale, which demands new detection technologies. Related fields that will also be impacted by quantum sensing are gravitational wave cosmology, astrophysics and fundamental tests of quantum mechanics. Quantum computing, along with traditional high-performance computing and advances in machine learning and artificial intelligence, will be absolutely necessary to analyse HL-LHC data. Quantum communication is also key to this.
What can high-energy physics contribute to quantum technologies?
Bringing the unique resources and expertise of the particle-physics community to bear on the development of quantum sensors will lead to rapid technology advances. For example, Fermilab develop high-Q superconducting RF cavities. Some searches for ultra-light dark matter use these.
Our leaders are not generals or CEOs, but colleagues called to serve for a time before returning to the rank and file
Additionally, they provide a high-coherence environment for qubits used as detectors, isolating them from a noisy environment. CERN, as the premier particle-physics laboratory in the world, will also find ways to contribute. In quantum sensing, CERN can help with its deep shafts potentially suited to atom interferometry. Several fledgling efforts exist, and collaboration can be enhanced by structures and funding and a world lab that brings people together from a wide range of disciplines.
How has becoming profoundly deaf at the age of 29 affected your career?
I was eight-months married and had just been appointed assistant professor when suddenly I fell very ill and was diagnosed with a rare cancer of the blood and bone marrow called acute myeloid leukemia, which few people at that time survived. I underwent intense chemotherapy, which weakened my immune system and caused me to fall into a coma. The hair cells in my cochlea were destroyed as a result of the antibiotics that were medically necessary to keep me safe until my own immune system had returned, rendering me permanently deaf. I was taught to lip read but I didn’t learn to sign because in general physics is not a culture where it is used. I also didn’t develop deaf speak. However, without hearing it was a slow process to communicate. There was immense support from my colleagues at Cornell and Persis Drell, who is now Provost at Stanford, was essential in taking it to the next level because she suggested she write down what people said. Others quickly followed suit, allowing me to communicate instantly for the first time. In 2003 I had a cochlear implant installed. When I couldn’t hear, I was treated completely like everyone else. I didn’t sense any discrimination. It taught me to be positive and to believe in myself and in life. Belief is important in everything we do both as individuals and as scientific institutions. Believing a 100 km circumference future circular collider is possible is a prerequisite for it to happen – and I believe!