This article from July 1969, written by the leader of the Electromagnetism Studies and Applications group, F. Rohrbach, covered the research carried out at CERN and elsewhere on the subject and future of very high voltage in vacuum.
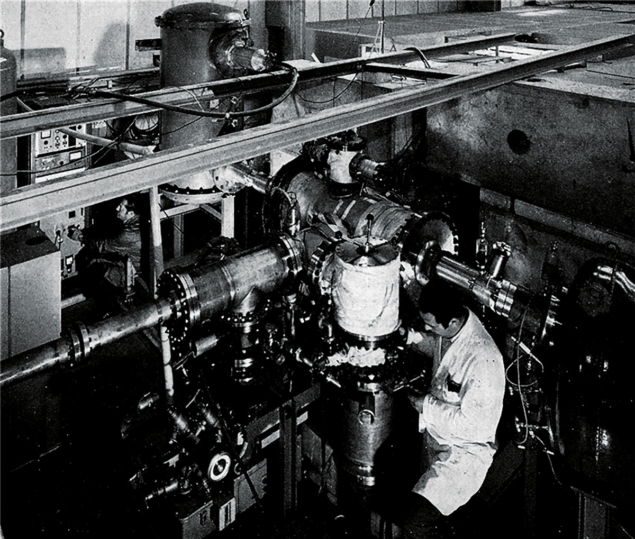
Whether it be in accelerators (accelerating columns, electrostatic inflectors, r.f. cavities or fast ejection units), secondary beams (separators and deflectors) or detectors (all types of spark chamber), the technological problems to be solved often centre around the insulation or switching of high voltages. In the case of insulation, attempts are made to minimize leakage currents, to eliminate flashover, to increase electric field gradients, or to lengthen the useful life and operational reliability. In the case of electrical breakdown, the salient factors are certain features of the arc such as jitter, rise time, lifetime, etc. for spark gaps; and brilliance, plasma size, memory, etc. for spark chambers and especially streamer chambers.
Over the past ten years, a great deal of work has been done, particularly in the USA and the USSR, on the insulation of high voltage in vacuum and on the physics of arcs, because of the rapid development of novel applications for high voltages – including X-ray flash tubes (operating at several MeV), circuit breakers, very high voltage electron microscopy (MV range), and equipment used in plasma physics and in high energy physics. Vacua, and even ultra high vacua, are involved in most of these new applications.
In 1964, the Massachusetts Institute of Technology, together with the University of Illinois and industry (the Ion Physics Corporation and the High Voltage Engineering Company), organized the first International Symposium on High Voltage Insulation in Vacuo. Currently these symposia take place every two years and are attended by delegates from all over the world. An international committee, on which CERN is represented, has been set up.
Since 1961, CERN has devoted special attention to this subject by stimulating applied research in connection with the development of electrostatic separators (see for example CERN COURIER vol. 9, page 132) and accelerating columns.
Technical developments with d.c. voltages
In spite of some spectacular fundamental discoveries concerning the physics involved, and some notable technological advances, the theory of breakdown in vacuum at very high voltage has still to be formulated. The most important technological advances, in which CERN has often played a pioneering role, include:
- Considerable improvements in voltage holding by abandoning stainless steel for the cathode in favour of heated glass (Berkeley), aluminium oxide (CERN) or titanium (CERN).
- Discovery of the marked effect of pressure and of the nature of the residual gas between 10~5 and 10–3 torr on behaviour under voltage and on operating life (many Laboratories including CERN).
- Discovery of the importance of the cleanliness of the surfaces subjected to powerful electric fields leading to the use of ultra high vacuum techniques (CERN).
The progress in the last ten years with homogeneous fields using large electrodes of the order of a square metre has made it possible to pass from 55–60 kV/cm over 5 cm and 40–50 kV/cm over 10 cm in 1960, to 150–160 kV/cm over 5 cm and 100–110 kV/cm over 10 cm in 1969. Nevertheless this is still far from the theoretical limit set by the field emission – 100 000 kV/cm!
The state of the theory
The large difference between the values obtained and the theoretical limit can now be explained. Though no new results have been published for some years on technical aspects, there have been some fundamental discoveries throwing new light on the mechanisms at the origin of an electric arc in vacuum. It has been found that there is high local amplification of the electric field at microscopic points which appear on metal surfaces under the action of intense fields. The heights of these points vary between a few tenths and several hundreds of microns. They can occur at either the anode or the cathode, but exactly how they are produced is still completely unknown.
It is now believed that there are several mechanisms which give rise to breakdown, the predominant one depending on parameters such as distance, voltage, residual pressure and the surface state of the electrodes. There are essentially two major regimes:
1. Short gaps between electrodes (less than a few millimetres) in a uniform field, or strong electric fields which are non-uniform (point-plane geometry);
2. Large gaps (more than a few millimetres) and very high voltages (more than a few hundred kV).
1. In the first regime, breakdown follows local heating either at the cathode due to field emission at the points which mysteriously develop, or at the anode by electron bombardment. The heating causes serious vaporization when current densities reach critical values between 107 and 108 A/cm2. The metal vapour thus produced is then rapidly ionized by cold emission electrons, leading to the final breakdown within a period varying from a few nanoseconds to a few hundred nanoseconds.
There have been some fundamental discoveries throwing new light on the mechanisms at the origin of an electric arc in vacuum
As the breakdown threshold is closely related to a critical current, and thus to a field, the characteristic breakdown voltage Vs as a function of the gap d between the electrodes should be linear. Also, the law of the variation of current with field should follow the predictions of field emission theory. These results have been confirmed over the past few years up to distances of a few millimetres between electrodes in a uniform, d.c, pulsed or high frequency field. The improvements in behaviour under pulsed voltage that can possibly be gained in this case are very small, when the time for which the voltage is applied is longer than a few tens of nanoseconds.
2. At CERN, with only a few exceptions, most of the applications of high voltage in vacuum are in the second regime which it had been difficult to study in University research laboratories because of the cost involved. Theoretical studies, in conjunction with experiments, were undertaken at CERN and have led to several new experimental observations and the elaboration of a model of the discharge phenomena.
When voltages are increased beyond a few hundred kV, the behaviour of the breakdown voltage threshold as a function of the different parameters changes completely. The characteristic Vs as a function of d is no longer linear but proportional to the square root of d. The residual pressure is of considerable importance (which is not so with short distances) and the threshold Vs is no longer determined by a critical current – the current before breakdown varies by several orders of magnitude when the distance varies only by a factor of two or three. Finally, and this is a fundamental point, the average time-lag to breakdown lengthens considerably – in the range of microseconds to several milliseconds.
These characteristics can be explained by the ‘micro-particle’ hypothesis. The mechanism leading to breakdown could then be described as the following: a collection of atoms is torn away from the anode as a result of the application of the field and electron bombardment. This micro-particle, electrically charged, is accelerated by the field between the electrodes and strikes the cathode with a velocity v and an energy W. If v and W are higher than critical values vc and Wc, the energy dissipated at the moment of striking is high enough, and remains within the interaction volume for long enough, to give rise to intense vaporization. Breakdown can then take place inside the bubble of gas thus formed. It can be shown by the double condition W greater than Wc and v greater than vc that the characteristic Vs as a function of d is then indeed of the square root form and that the minimum time lag T min is such that In T min is linear with V2.
Application of the theory to pulsed voltages
For high voltages (MV) and large distances (cm) in ultra high vacua (10~9 to 1CT8 torr), present investigations at CERN show that, in fact, the mechanism involved in initiating a breakdown takes a considerable time (jis to ms) to develop and that these times are statistically distributed in such a way that T min is proportional to V2. Because of these results, obtained with stainless steel and titanium electrodes, it would be possible to increase the strength of electric fields in vacuum very appreciably for all applications where the field is needed for only a short time (a maximum of several microseconds), which is often the case around large particle accelerators. The advantages obtained would allow the present values of d.c. fields to be doubled for times of the order of a few microseconds and distances between electrodes greater than a centimetre.
Future possibilities
Such an increase in the intensity of electric fields would allow further steps forward in the use of high energy particle separators and fast deflectors. Other conceivable applications include strong field accelerating lines in electron ring accelerators, coaxial beam guides, electromagnetic lenses, etc.
In all these applications, the new technical problem which arises is that of generating voltage pulses of several MV with very steep leading edges (less than 10 ns). The duration of the pulse depends on the application in view (from 10 ns to a few lis). A Marx generator in conjunction with a Blumlein line can be used for very short pulses as is already done for the Stanford 600 kV streamer chamber (see CERN COURIER vol. 7, page 219). The most delicate problems are those involved in striking the main spark-gap in the Blumlein line with a very low jitter (ns), since this spark-gap must operate at 2 MV with an impedance of 30 ohms. There are thoughts of using a ruby laser, a multiple-electrode spark-gap or perhaps a liquid dielectric spark-gap. A rise time of 50 to 100 ns would be adequate for pulses lasting several mircroseconds, in which case the Blumlein line would not be needed.
Many other laboratories and commercial firms are particularly interested in the work currently being done at CERN in the field of high voltages
In an application of the deflector or separator type, the beam element can be included in the load on the Blumlein line. Profit could then be drawn from having the magnetic field in phase with the electric field, and a TEM (transverse electromagnetic) wave is then set up. There are two advantages:
1. If the particles are sent into the equipment in the same direction as that of the propagation of the wave, the unit is an electromagnetic separator – a velocity selector with automatic magnetic compensation (with chromatic aberrations reduced to a minimum).
2. If the particles are sent in the opposite direction to the TEM wave, then the electric and magnetic deflections are added together and an electromagnetic deflector is formed. In view of the present technical results, deflectors can be made with 450 to 500 kV/cm (1 MV over 2 cm), or the equivalent of 3 kG. Thus the same piece of equipment can serve either as a deflector or as a separator.
The main potential of high electric field electromagnetic separators lies in the field of the separation of low-energy (a few hundred MeV) kaon beams for bubble chambers. It is possible in these cases to reduce the length of the separator considerably while retaining the same angle of separation, and thus to have particle beams with a short decay length (0.75 m for 100 MeV charged kaons).
The refined technology which has been developed to obtain very high voltage pulses and to overcome the complex problems in striking a spark-gap operating at several MV, allow one to think of building streamer chambers with very high gaps (of the order of a metre) using such high voltages. It is probably in this direction that interest in pulsed high voltages will be concentrated, because the advantages offered by such chambers are so attractive that physicists will almost certainly want them built. Finally, it is interesting to note that many other laboratories and commercial firms are particularly interested in the work currently being done at CERN in the field of high voltages. These laboratories and firms include those working in such varied fields as emitters, the transmission of electric power, circuit breakers, rectifiers, colour television, etc…
- This article was adapted from text in CERN Courier vol. 9, July 1969, pp208–210