Having successfully fulfilled its mission to track down the quark contribution to the nucleon’s spin, HERMES now aims at providing an even more complete picture of nucleon spin.
At the end of the 1980s, a major part of the nucleon’s spin suddenly went missing. The European Muon Collaboration at CERN uncovered what has since been called the “spin puzzle” – the fact that the spins of the valence quarks that make up the nucleon account for only about 25-30% of the nucleon’s spin. The finding was soon confirmed by second-generation experiments at CERN and SLAC. Designed to determine the total spin contribution of the quarks, however, they left several questions unanswered. If the quark spin contribution is so small, what then are the main contributions? What is the contribution of the different quark flavours? Is there a polarization in the quark sea that could account for the missing spin? In 1995, the HERMES experiment at DESY’s HERA electron-proton collider in Hamburg took over the search, with the goal of finding an answer to just these questions.

In contrast to its predecessors, most of which could detect only the scattered electron and thus measure inclusive deep inelastic scattering (DIS) reactions, the HERMES collaboration took a new experimental approach. Its combination of a longitudinally polarized high-energy electron beam from the HERA storage ring incident on undiluted polarized atomic gas targets is unique in the field, and its spectrometer is designed to identify all types of hadrons produced in coincidence with the scattered electron. Using such semi-inclusive DIS reactions on longitudinally polarized targets, the HERMES team achieved the world’s first assumption-free flavour separation of the quark contributions to the nucleon spin, thereby slotting a major new piece into the nucleon-spin puzzle.

The results obtained from data taken during HERMES’ first run (1995-2000) are the most precise information available so far on quark helicity distributions – the spin alignment of the quarks with respect to the nucleon spin – and they provide for the first time separate determinations of the polarizations of the up, down and strange sea quarks (figure 3). They reveal that the largest contribution to the nucleon spin comes from the valence region, where the up quarks give a positive contribution as their spin is preferably aligned with the spin of the nucleon, while the down quarks give a contribution with opposite sign. The polarizations of the sea quarks are all consistent with zero – an especially important result.

The interpretation of the inclusive data from previous experiments was based on the assumption of SU(3) flavour symmetry – the postulation that all flavours of quark, including up, down and the virtual strange quarks, behave in the same way dynamically inside the nucleon despite the substantially greater mass of the strange quark. The older analyses therefore led to the conclusion that the strange quarks play a significant, cancelling, role in the nucleon spin, even though their existence there is fleeting. The HERMES results now show that the polarizations of the sea quarks are all small: there is thus little evidence for such a “cancellation” between the contributions of valence and sea quarks. In particular, there is no evidence in the measured range in x, the momentum fraction carried by the quarks, that the contribution of the strange quarks is negative, as was indicated by the model-dependent analysis.
Taken together, all these measurements definitely show that the spin of the quarks generates less than half of the spin of the nucleon, and that the quark spins that do contribute come almost exclusively from the valence quarks. The proton-spin puzzle thus continues to evolve and the contributions of the orbital angular momenta of the quarks, as well as the spins and orbital angular momenta of the gluons, are now expected to be important.
The HERMES team has already reported the first evidence that the gluon polarization could make a positive contribution to the nucleon spin and new results will follow soon. The gluon polarization is also currently being investigated in the COMPASS experiment at CERN and at the Relativistic Heavy Ion Collider at the Brookhaven National Laboratory. As recent theoretical insights have shown, the orbital angular momentum can in principle be probed by hard exclusive processes leaving the target nucleon intact – a field that HERMES is now exploring in detail during its ongoing second run, which will last until summer 2007.
During HERMES’ first run, the emphasis was on the determination of the helicity structure of the nucleon using longitudinally polarized targets and beam. For HERA Run II, the collaboration has turned its attention to transversely polarized targets (i.e. with polarizations perpendicular to the electron beam direction) to extract the so-called transversity distribution – the last unknown leading-twist quark distribution function of the nucleon.
Probing transversity
Phenomenologically, the nucleon can be characterized in terms of parton distribution functions that describe how often the constituents of the nucleon will be found in a certain state. Within this framework, there are three fundamental quark distributions: the quark number density, which has been measured with very high precision, for example with the HERA collider experiments H1 and ZEUS; the helicity distribution, which was the main result of the HERMES run on longitudinally polarized targets; and the transversity distribution, which describes the difference in the probabilities to find quarks in a transversely polarized nucleon with their spin aligned to the spin of the nucleon and quarks with their spin anti-aligned.
In absence of relativistic effects, the transversity and helicity distributions should be the same. A difference between the two distributions would therefore be a measure of the extent to which relativistic effects have to be considered in the description of the nucleon.
Transversity has remained unmeasured so far because it is odd under chirality transformations, whereas hard interactions conserve chirality (they are chiral even). However, it may be probed by a process involving some additional chiral-odd structure. In semi-inclusive DIS, as done at HERMES, this could be a chiral-odd fragmentation function. The so-called Collins fragmentation function is the most prominent candidate, as this gives rise to an asymmetry in the angular distribution of the hadrons produced during the scattering process if they are generated from a transversely polarized quark. Results from the BELLE collaboration at KEK suggest that the Collins function has a substantial magnitude, and thus measurements of single-spin asymmetries in semi-inclusive DIS employing transverse target polarization are expected to constrain transversity itself.
There is even more to explore, however. Using a transversely polarized target one can also study a new class of more complex distribution functions that depend not only on the longitudinal momentum fraction carried by the quarks, but also on their transverse momentum inside the nucleon. One such function is the so-called Sivers distribution function, which describes an asymmetry in the distribution of unpolarized quarks in a transversely polarized nucleon.
The Sivers function generates transverse single-spin asymmetries. As such it is a so-called T-odd distribution function. The time-reversal symmetry properties of quantum chromodynamics should therefore forbid its existence, or so it was believed for a long time. Only recently has it become clear that there are loopholes in the theory, for example, missing gauge links in the description of nucleon structure, which permit such T-odd distribution functions. The study of the Sivers function is thus doubly interesting. On one hand, it may be used to test the validity of these theoretical considerations, and may lead to a better understanding of results from hadron-hadron collision experiments where large but unexplained transverse single-spin asymmetries have been observed. On the other hand, the Sivers function must vanish in the absence of quark orbital angular momentum. A measurement of the Sivers function may thus provide important constraints on this missing piece in the nucleon-spin puzzle.
The measurement both of transversity and of the Sivers function can be carried out using semi-inclusive DIS events. By reversing the spin direction of the nucleon, one can study the dependence of the preferred direction of the outgoing hadron on the nucleon’s spin direction. After its first year of data-taking with a transversely polarized target, HERMES has observed enough scattering events of this kind to venture a worldwide first look at these angular dependencies (figure 4).
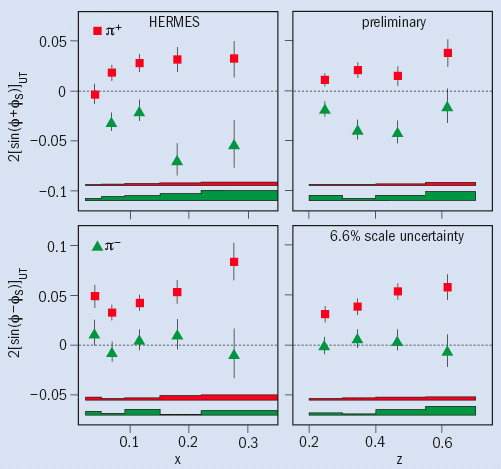
One striking result is the comparably large signal for the π– Collins effect. This came as quite a surprise, since a behaviour similar to that seen with a longitudinally polarized target had been expected, namely a signal that is larger in size for the π+ than for the π–. This result will have important consequences for understanding how the hadron is formed from the struck quark. Currently the statistical significance is insufficient to make definite statements about the exact behaviour of the involved functions. However, since the Collins asymmetries are clearly non-vanishing, the HERMES team has good reason to hope that the data collected in 2005, which are more than doubling the statistics taken in the years before, will indeed allow them to achieve a first measurement of transversity using the information on the Collins fragmentation function collected by BELLE.
An equally interesting result is the observation that for the π+ the signal for the Sivers function is significantly positive. This is the first confirmation that the Sivers function is indeed non-zero and thus some first evidence for a non-vanishing T-odd parton distribution function. More data on the Sivers asymmetries from HERMES up to November 2005 should allow an extraction of the Sivers function for up and down quarks.
Exclusive reactions
The contributions of the orbital angular momenta of quarks and gluons are the last unknown pieces in the puzzle of the spin content of the nucleon; how to determine them has been hotly debated for several years. In the middle of the 1990s, it was realized that the framework of generalized parton distributions (GPDs) might give access to these orbital angular momentum contributions. Quite apart from that, the study of these off-forward extensions of the standard parton distribution functions can provide a wealth of new information on the structure of the nucleons.
The GPDs can be determined in exclusive reactions – scattering processes in which the target nucleon does not fragment but instead remains in its ground state, or close to it. The various scattering reactions provide access to different GPDs: exclusive vector-meson production allows the determination of unpolarized GPDs, whereas the exclusive production of pseudoscalar mesons can be used to measure the polarized GPDs. Because of the multidimensional structure of the GPDs, it is essential to study as many different processes as possible to be able to disentangle the functions from the measured observables. In particular, measurements with the transversely polarized target at HERMES are sensitive to the two main GPDs (H and E) necessary to determine Jq, the total orbital angular momentum of quarks in the nucleon.
The cleanest example of a reaction that provides direct access to GPDs is deeply virtual Compton scattering (DVCS), an exclusive reaction in which a real photon is created. This type of reaction was identified for the first time in 2001 by the H1, ZEUS and HERMES experiments at DESY, and the CEBAF Large Angle Spectrometer in Hall B at Jefferson Lab. Since then, a large body of data has been collected on DVCS. Here, HERMES is in a rather fortunate position. Despite the DVCS cross-section being usually much lower than that of an indistinguishable process – the Bethe-Heitler process – with identical final state, the interference term between the processes allows the study of DVCS because it leads to observable asymmetries in the azimuthal angular dependence of the real photons produced from the nucleon. As HERMES can measure all azimuthal asymmetries involving polarized beams and/or polarized targets and different charges, the collaboration was able to determine the DVCS target-spin asymmetry by using an unpolarized beam incident on a transversely polarized target. This asymmetry is sensitive to the GPD E and thus allowed HERMES to realise the first model-dependent extraction of Ju, the total angular momentum of the up quark (figure 5).
To study these asymmetries in even more detail, the HERMES collaboration decided to run during its final phase from 2006 to 2007 with a new recoil detector in combination with an unpolarized target. The device will employ silicon detectors and scintillating fibre trackers as well as a detector to measure photons. It will sit closely around the target and detect the slow-moving recoil proton in coincidence with the electron and the photon, thus ensuring full exclusivity of the data sample.
The enhanced selectivity of these measurements will provide a unique opportunity to assess the promise of GPDs as the next step in understanding the spin structure of the nucleon. In particular, data taken with this new detector will put serious constraints on the GPD H. In combination with the already existing DVCS data and measurements of the only other known reaction to access the GPD E on a proton target – the elastic electroproduction of ρ0 vector mesons with the proton being transversely polarized, already measured at HERMES (2002-2005) – these forthcoming results will eventually allow an extraction of the total angular momentum of the up quark in the nucleon through the remaining GPD models.
In addition to these studies on polarized targets, the physics programme at HERMES also includes a great variety of other points of interest, such as measurements of unpolarized DIS events. These allow the collaboration to search for pentaquark exotic baryon states, give insight into quark propagation in nuclear matter and quark fragmentation, and provide a rigorous test of factorization. DESY’s HERA accelerator will continue operations through to summer 2007. Every effort is being made at HERMES to maximize the impact of the remaining beam time, with the goal of fitting in as many pieces of the nucleon-spin puzzle as possible.
Further reading
The HERMES website is at www-hermes.desy.de.
A Airapetian et al. 2005a Phys. Rev D 71 012003.
A Airapetian et al. 2005b Phys. Rev. Lett. 94 012002.
Z Ye 2005 http://arXiv.org/abs/hep-ex/0512010; to appear in the proceedings of the International Europhysics Conference on High Energy Physics, 21-27 July 2005, Lisboa, Portugal.