An experiment at the Stanford Linear Accelerator Center has recently focused positron beams by means of a plasma lens. This is the first time this process has been observed.
In colliding beam physics, the luminosity (collision rate) of a machine determines the yields of interesting high -energy events. Future linear colliders could significantly benefit if the colliding beams were additionally focused to a smaller transverse size beyond that which is possible with conventional or superconducting magnetic beam transport elements.
The theory of a self-focusing plasma lens was first proposed in 1987 by Pisin Chen who also leads the current experiment at the Stanford Linear Accelerator Center (SLAC). If such lenses were, for example, located at the interaction point of a collider, they could focus both the electron and positron beams, thereby reducing beam spot size and increasing the luminosity, perhaps by one order of magnitude or more.
The Experiment E-150 Plasma Lens Collaboration was formed to investigate this process and study the feasibility of its application at proposed future linear colliders like the Next Linear Collider. The collaboration contains members from four laboratories and three universities (see the complete list at the end of this article).
Gas
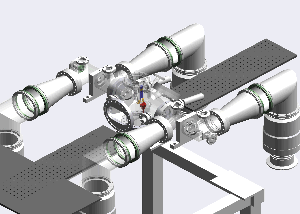
The process started with a positron beam from the SLAC PEP-II positron source. This was sent through a damping ring and then accelerated to 28.5 GeV in the SLAC linac with a bunch intensity of 1-2 x 1010. The beam was delivered to the Final Focus Test Beam Facility (FFTB) at a rate of 1 or 10 Hz. At the focal point of the FFTB transport, a special plasma chamber contains a 3 mm diameter pulsed gas nozzle (see diagram) through which either hydrogen or nitrogen gas is “puffed” into the ultrahigh vacuum system at plenum gas pressures up to 75 atm with a discharge time of 800 ms. The gas is pumped off by a Roots-type pump. On either side of the central chamber are differential pumping sections semi-isolated from each other by thin titanium windows with small (2-5 mm diameter) apertures for the positron beams to pass through. These sections are evacuated by turbomolecular pumps and allow operation of the plasma lens with ultrahigh vacuum systems on either side.
The plasma lens was generated by ionizing the gas using a pulsed YAG laser operating at 10 Hz in the infrared region (wavelength 1064 nm) and delivering a pulse energy of 1.5 J. The relativistic positron beam exhibits effects from both its charge and its current. For a beam propagating in a vacuum, the Lorentz force induced by the collective electric and magnetic fields is nearly cancelled, which is why a high-energy beam can travel over kilometres without much increase in spread (emittance).
Plasma response
Upon entering an initially quiescent plasma, the plasma response to the intruding charge and current is such that the plasma electrons are attracted into the positron beam so as to neutralize the space charge of the beam and thereby cancel its radial electric field, which tends to self-repel the positrons. However, if the beam radius is much smaller than the natural plasma wavelength, the neutralization of the intruding beam current by the plasma return current is ineffective. This leaves the azimuthal magnetic field unbalanced, and this field focuses the beam.
The plasma lens concept also works for electron beams. In that case, the plasma electrons are expelled from the beam volume. The result is a near uniform focusing of the beam due to the less mobile ions – the beam is “pinched”.
In the FFTB experiment, typical plasma densities were of the order of 1018/cm3, corresponding to a plasma wavelength of about 30 mm. This is indeed much larger than the incoming beam radius, which is about 5 mm.
The focusing strength of such a lens is equivalent to that of focusing magnets like quadrupoles with gradients of the order of 106 T/m. For comparison, a conventional small aperture (1 cm diameter) iron core quadrupole can maximally be excited to about 250 T/m.
The focusing effect of the plasma lens was measured using proven wire scanner technology developed for the SLC and FFTB. The wires are 4 mm and 7 mm carbon fibres. The scanner is located just downbeam of the plasma lens and is adjustable to allow mapping of the transverse beam size and pinpointing the longitudinal location of the beam waist.
Measuring the beam
The bremsstrahlung photons emitted by the positron scattering off the wires are detected in a Cherenkov cell type detector located 33 m downbeam of the lens. The variation in photon yield as the beam “dithers” across the wire provides a measure of the transverse beam profile from which the beam size can be determined.
A second, independent method to measure the strength of the plasma lens is a segmented synchrotron radiation monitor some 35 m downbeam of the lens. The harder the beam is focused, the higher the energy of the emitted synchrotron radiation. As the plasma focusing effect is transient, the monitoring of this synchrotron radiation provides an “on-line” measurement of the plasma focusing gradient. Such a plasma focusing induced synchrotron radiation signal was also observed for the first time. The energy was determined to be a few mega-electron-volts, which confirms the gradients derived from the plasma density.
Smaller beams
Figure 1 shows a typical set of scans for hydrogen and nitrogen (Z is in the direction of the momentum vector of the beam), illustrating the beam’s convergence toward a waist.
The plasma lens concept also works if there is no pre-ionization by the laser, a process called self or impact ionization. Here, the head of the positron bunch ionizes the gas and the remainder of the bunch is focused. The head of the bunch is not focused, so the efficiency in spot size reduction is lower than for laser pre-ionization. For the latter, the beam size was approximately halved for nitrogen. The reduction in the orthogonal dimension was comparable, so that the reduction in spot size was approximately a factor of four. The maximum possible spot size decrease is much higher, but in the SLAC experiment, the beam current had to be lowered and the incoming beam size enlarged so the fragile carbon fibres in the wire scanner would not melt in the very-high-energy density of the focused beam.
Earlier, the experimental setup was used to focus a 30 GeV electron beam using the self-ionization method. The collaboration is excited about potential future applications of the plasma lens concept and is presently repeating this electron focusing experiment using also laser pre-ionization.
The members of the collaboration, by institution, are: Fermilab (C Crawford and R Noble); KEK-Japan (K Nakajima); Lawrence Livermore (H Baldis and P Bolton); SLAC (P Chen, W Craddock, F-J Decker, C Field, R Iverson, F King, RKirby, J Ng, P Raimondi and D Walz); Hiroshima University, Japan (A Ogata), UCLA (D Cline, Y Fukui and V Kumar); University of Tennessee (A Weidemann).