The European Spallation Source (ESS) will provide neutron beams 100 times brighter than those from reactor sources, enabling new research into material properties and fundamental physics.
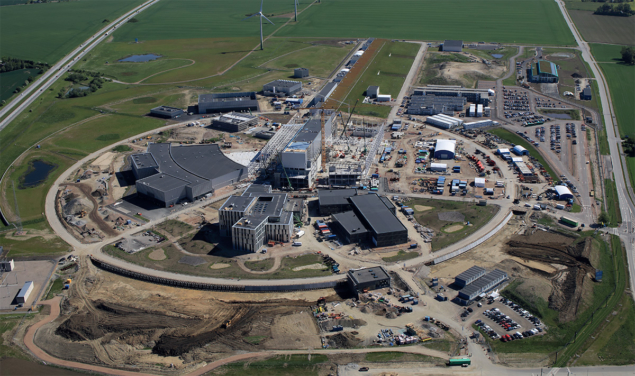
Just a few years after the discovery of the neutron by James Chadwick in 1932, investigations into the properties of neutrons by Fermi and others revealed the strong energy dependence of the neutron’s interactions with matter. This knowledge enabled the development of sustainable neutron production by fission, opening the era of atomic energy. The first nuclear-fission reactors in the 1940s were also equipped with the capacity for materials irradiation, and some provided low-energy (thermal) neutron beams of sufficient intensity for studies of atomic and molecular structure. Despite the high cost of investment in nuclear-research reactors, neutron science flourished to become a mainstay among large-scale facilities for materials research around the world.
The electrical neutrality of neutrons allows them to probe deep into matter in a non-destructive manner, where they scatter off atomic nuclei to reveal important information about atomic and molecular structure and dynamics. Neutrons also carry a magnetic moment. This property, combined with their absence of electric charge, make neutrons uniquely sensitive to magnetism at an atomic level. On the downside, the absence of electric charge means that neutron-scattering cross sections are much weaker than they are for X-rays and electrons, making neutron flux a limiting factor in the power of this method for scientific research.

Throughout the 1950s and 1960s, incremental advances in the power of nuclear-research reactors and improvements in moderator design provided increasing fluxes of thermal neutrons. In Europe these developments culminated in the construction of the 57 MW high-flux reactor (HFR) at the Institut Laue-Langevin (ILL) in Grenoble, France, with a compact core containing 9 kg of highly enriched uranium enabling neutron beams with energies from around 50 μeV to 500 meV. When the HFR came into operation in 1972, however, it was clear that nuclear-fission reactors were already approaching their limit in terms of steady-state neutron flux (roughly 1.5 × 1015 neutrons per cm2 per second).
Spallation has long been hailed as the method with the potential to push through to far greater neutron fluxes
In an effort to maintain pace with advances in other methods for materials research, such as synchrotron X-ray facilities and electron microscopy, accelerator-based neutron sources were established in the 1980s in the US (IPNS and LANSCE), Japan (KENS) and the UK (ISIS). Spallation has long been hailed as the method with the potential to push through to far greater neutron fluxes, and hence to provide a basis for continued growth of neutron science. However, after nearly 50 years of operation, and with 10 more modern medium- to high-flux neutron sources (including five spallation sources) in operation around the world, the HFR is still the benchmark source for neutron-beam research. Of the spallation sources, the most powerful (SNS at Oak Ridge National Laboratory in the US and J-PARC in Japan) have now been in operation for more than a decade. SNS has reached its design power of 1.4 MW, and J-PARC is planning for tests at 1 MW. At these power levels the sources are competitive with ILL for leading-edge research. It has long been known that the establishment of a new high-flux spallation neutron facility is needed if European science is to avoid a severe shortage in access to neutron science in the coming years (CERN Courier May/June 2020 p49).
Unprecedented performance
The European Spallation Source (ESS), with a budget of €1.8 billion (2013 figures), is a next-generation high-flux neutron source that is currently entering its final construction phase. Fed by a 5 MW proton linac, and fitted with the most compact neutron moderator and matched neutron transport systems, at full power the brightness of the ESS neutron beams is predicted to exceed the HFR by more than two orders of magnitude.
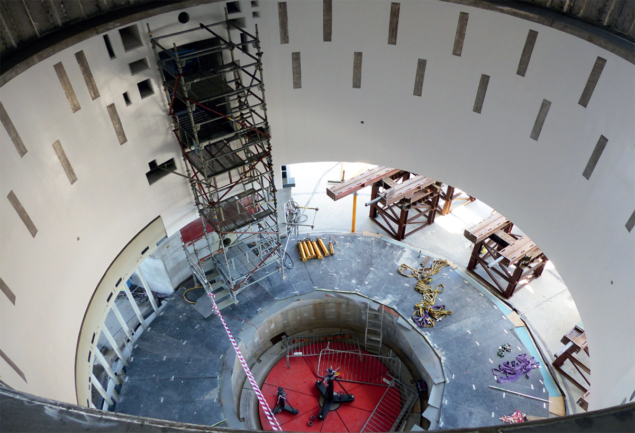
The idea for the ESS was advanced in the early 1990s. The decision in 2009 to locate it in Lund, Sweden, led to the establishment of an organisation to build and operate the facility (ESS AB) in 2010. Ground-breaking took place in 2014, and today construction is in full swing, with first science expected in 2023 and full user operation in 2026. The ESS is organised as a European Research Infrastructure Consortium (ERIC) and at present has 13 member states: Czech Republic, Denmark, Estonia, France, Germany, Hungary, Italy, Norway, Poland, Spain, Sweden, Switzerland and the UK. Sweden and Denmark are the host countries, providing nearly half of the budget for the construction phase. Around 70% of the funding from the non-host countries is in the form of in-kind contributions, meaning that the countries are delivering components, personnel or other support services to the facility rather than cash.
The unprecedented brightness of ESS neutrons will enable smaller samples, faster measurements and more complex experiments than what is possible at existing neutron sources. This will inevitably lead to discoveries across a wide range of scientific disciplines, from condensed-matter physics, solid-state chemistry and materials sciences, to life sciences, medicine and cultural heritage. A wide range of industrial applications in polymer science and engineering are also anticipated, while new avenues in fundamental physics will be opened (see “Fundamental physics at the ESS” panel).
Fundamental physics at the ESS
The ESS will offer a multitude of opportunities for fundamental physics with neutrons, neutrinos and potentially other secondary particles from additional target stations. While neutron brightness and pulse time structure are key parameters for neutron scattering (the main focus of ESS experiments), the total intensity is more important for many fundamental-physics experiments.
A cold neutron-beam facility for particle physics called ANNI is proposed to allow precision measurements of the beta decay, hadronic weak interactions and electromagnetic properties of the neutron. ANNI will improve the accuracy of measurements of neutron beta decay by an order of magnitude. Experiments will probe a broad range of new-physics models at mass scales from 1 to 100 TeV, far beyond the threshold of direct particle production at accelerators, and resolve the tiny effects of hadronic weak interactions, enabling quantitative tests of the non-perturbative limit of quantum chromodynamics.
Another collaboration is proposing a two-stage experiment at the ESS to search for baryon-number violation. The first stage, HIBEAM, will look for evidence for sterile neutrinos. As a second stage, NNBAR could be installed at the large beam port, with the purpose to search for oscillations between neutrons and anti-neutrons. Observing such a transition would show that the baryon number is violated by two units and that matter containing neutrons is unstable, potentially shedding light on the observed baryon asymmetry of the universe.
A design study, financed through the European Commission’s Horizon 2020 programme, is also under way for the ESS Neutrino Super Beam (ESSνSB) project. This ambitious project would see an accumulator ring and a separate neutrino target added to the ESS facility, with the aim of sending neutrinos to a large underground detector in mid-Sweden, 400–500 km from the ESS. Here, the neutrinos would be detected at their second oscillation maximum, giving the highest sensitivity for discovery and/or measurement of the leptonic CP-violating phase. An accumulator ring and the resulting short proton pulses needed by ESSνSB would open up for other kinds of fundamental physics as well as for new perspectives in neutron scattering, and muon storage rings.
Finally, a proposal has been submitted to ESS concerning coherent neutrino–nucleus scattering (CEνNS). The high proton beam power together with the 2 GeV proton energy will provide a 10 times higher neutrino flux from the spallation target than previously obtained for CEνNS. Measured for the first time by the COHERENT collaboration in 2017 at ORNL’s Spallation Neutron Source, CEνNS offers a new way to probe the properties of the neutrino including searches for sterile neutrinos and a neutrino magnetic moment, and could help reduce the mass of neutrino detectors.
From the start, the ESS has been driven by the neutron-scattering community, with strong involvement from all the leading neutron-science facilities around Europe. To maximise its scientific potential, a reference set of 22 instrument concepts was developed from which 15 instruments covering a wide range of applications were selected for construction. The suite includes three diffractometers for hard-matter structure determination, a diffractometer for macromolecular crystallography, two small-angle scattering instruments for the study of large-scale structures, two reflectometers for the study of surfaces and interfaces, five spectrometers for the study of atomic and molecular dynamics over an energy range from a few μeV to several hundred meV, a diffractometer for engineering studies and a neutron imaging station (see “ESS layout” figure). Given that the ESS target system has the capacity for two neutron moderators and that the beam extraction system allows viewing of each moderator by up to 42 beam ports, there is the potential for many more neutron instruments without major investment in the basic infrastructure. The ESS source also has a unique time structure, with far longer pulses than existing pulsed sources, and an innovative bi-spectral neutron moderator, which allows a high degree of flexibility in the choice of neutron energy.
Accelerator and target
Most of the existing spallation neutron sources use a linear accelerator to accelerate protons to high energies. The particles are stored in an accumulator ring and are then extracted in a short pulse (typically a few microseconds in length) to a heavy-metal spallation target such as tungsten or mercury, which have a high neutron yield. A notable exception is SINQ at PSI, which uses a cyclotron that produces a continuous beam.
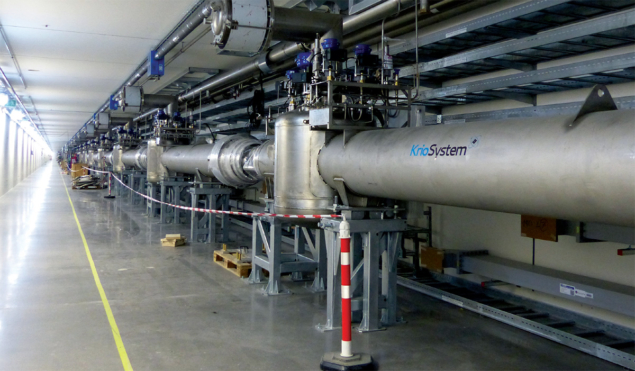
ESS has a linear accelerator but no accumulator ring, and it will thus have far longer proton pulses of 2.86 ms. This characteristic, combined with the 14 Hz repetition rate of the ESS accelerator, is a key advantage of the ESS for studies of condensed matter, because it allows good energy resolution and broad dynamic range. The result is a source with unprecedented flexibility to be optimised for studies from condensed-matter physics and solid-state chemistry, to polymers and the biological sciences with applications to medical research, industrial materials and cultural heritage. The ESS concept is also of major benefit for experiments in fundamental physics, where the total integrated flux is a main figure of merit.
The high neutron flux at ESS is possible because it will be driven by the world’s most powerful particle accelerator, in terms of MW of beam on target. It will have a proton beam of 62.5 mA accelerated to 2 GeV, with most of the energy gain coming from superconducting radio-frequency cavities cooled to 2 K. Together with its long pulse structure, this gives 5 MW average power and 125 MW of peak power. For proton energies around a few GeV, the neutron production is nearly proportional to the beam power, so the ratio between beam current and beam energy is to a large extent the result of a cost optimisation, while the pulse structure is set by requirements from neutron science.

The neutrons are produced by spallation when the high-energy protons hit the rotating tungsten target. The 2.5 m-diameter target wheel consists of 36 sectors of tungsten blocks inside a stainless-steel disk. It is cooled by helium gas, and it rotates at approximately 0.4 Hz, such that successive beam pulses hit adjacent sectors to allow adequate heat dissipation and limiting radiation damage. The neutrons enter moderator–reflector systems above or below the target wheel. The unique ESS “butterfly” moderator design consists of interpenetrating vessels of water and parahydrogen, and allows viewing of either or both vessels from a 120° wide array of beam ports on either side. The moderator is only 3 cm high, ensuring the highest possible brightness. Thus each instrument is fed by an intense mix of thermal (room temperature) and cold (20 K) neutrons that is optimised to its scientific requirements. The neutrons are transported to the instruments through neutron-reflecting guides that are up to 165 m long. Neutron optics are quite challenging, due to the weak cross-sections, which makes the technology for transporting neutrons sophisticated. The guides consist of optically flat glass or metal channels coated with many thin alternating layers of nickel and titanium, in a sequence designed to enhance the critical angle for reflection. The optical properties of the guides allow for broad spectrum focusing to maximise intensity for varying sample sizes, typically in the range from a few mm3 to several cm3.
Under construction
Construction of the ESS has been growing in intensity since it began in 2014. The infrastructure part was organised differently compared to other scientific large-scale research facilities. A partnering collaboration agreement was set up with the main contractor (Skanska), with separate agreements for the design and target cost settled at the beginning of different stages of the construction to make it a shared interest to build the facility within budget and schedule.
Every year, up to 3000 researchers from all over the world are expected to carry out around 1000 experiments
Today, all the accelerator buildings have been handed over from the contractor to ESS. The ion source, where the protons are produced from hydrogen gas, was delivered from INFN in Catania at the end of 2017. After installation, testing and commissioning to nominal beam parameters, the ion source was inaugurated by the Swedish king and the Italian president in November 2018. Since then, the radio-frequency quadrupole and other accelerator components have been put into position in the accelerator tunnel, and the first prototype cryomodule has been cooled to 2 K. There is intense installation activity in the accelerator, where 5 km of radio-frequency waveguides are being mounted, 6000 welds of cooling-water pipes performed and 25,000 cables being pulled. The target building is under construction, and has reached its full height of 31 m. The large target vacuum vessel is due to arrive from in-kind partner ESS Bilbao in Spain later this year, and the target wheel in early 2021.
The handover of buildings for the neutron instruments started in September 2019, with the hall of the long instruments along with the buildings housing associated laboratories and workshops. While basic infrastructure such as the neutron bunker and radiation shielding for the neutron guides are provided by ESS in Lund, European partner laboratories are heavily involved in the design and construction of the neutron instruments and the sample-environment equipment. ESS has developed its own detector and chopper technologies for the neutron instruments, and these are being deployed for a number of the instruments currently under construction. In parallel, the ESS Data Management and Software Centre, located in Copenhagen, Denmark, is managing the development of instrument control, data management and visualisation and analysis systems. During full operation, the ESS will produce scientific data at a rate of around 10 PB per year, while the complexity of the data-handling requirements for the different instruments and the need for real-time visualisation and processing add additional challenges.

The major upcoming milestones for the ESS project are beam-on-target, when first neutrons are produced, and first-science, when the first neutron-scattering experiments take place. According to current schedules, these milestones will be reached in October 2022 and July 2023, respectively. Although beam power at the first-science milestone is expected to be around 100 kW, performance simulations indicate that the quality of results from first experiments will still have a high impact with the user community. The initiation of an open user programme, with three or more of the neutron instruments beginning operation, is expected in 2024, with further instruments becoming available for operation in 2025. When the construction phase ends in late 2025, ESS is expected to be operating at 2 MW, and all 15 neutron instruments will be in operation or ready for hot-commissioning.
The ESS has been funded to provide a service to the scientific community for leading-edge research into materials properties. Every year, up to 3000 researchers from all over the world are expected to carry out around 1000 experiments there. Innovation in the design of the accelerator, the target system and its moderators, and in the key neutron technologies of the neutron instruments (neutron guides, detectors and choppers), ensure that the ESS will establish itself at the vanguard of scientific discovery and development well into the 21st century. Furthermore, provision has been made for the expansion of the ESS to provide a platform for leading-edge research into fundamental physics and as yet unidentified fields of research.
Further reading
K H Andersen et al. 2020 Nucl. Instrum. Methods Phys. Res. A 957 163402.
D Baxter et al. 2019 arXiv:1911.00762.
R Garoby et al. 2018 Phys. Scr. 93 014001.
D Milstead 2015 arXiv:1510.01569.
T Soldner et al. 2019 EPJ Web Conf. 219 10003.
E Wildner et al. 2016 Adv. High Energy Phys. 2016 8640493.