Ways to increase physics potential and luminosity at Brookhaven’s collider.
Discoveries at RHIC, at the Brookhaven National Laboratory (BNL), have captured worldwide attention. These findings have raised compelling new questions about the theory that describes the interactions of the smallest known components of the atomic nucleus. To address these questions at RHIC, we need to study rare processes. To do this, we must increase the collider’s luminosity, which is the rate at which ions collide inside the accelerator. BNL’s Collider-Accelerator Department (C-AD) is therefore investigating various upgrades, including the possibility of a luminosity upgrade through a process of electron cooling.
The electron-cooled RHIC, known as RHIC-II, would use low-emittance (“cool”), energetic and high-charge bunches of electrons to cool the ion bunches. This would increase the density of the ion bunches and lead to a higher luminosity. Achieving the necessary characteristics for the electron bunches will require using advanced accelerator techniques such as a high-brightness, high-current energy-recovery linac (ERL). A linac of this type may have other applications, including in an eRHIC (energetic electron ion collider at RHIC) and future light sources.
As RHIC operates, its luminosity goes down because of intra-beam scattering (IBS), which causes the bunches of gold ions to increase their longitudinal emittance and transverse emittance. This means that the bunches “heat up” and become more diffuse. A variety of other mechanisms can also induce emittance growth, regardless of IBS. These include instabilities of the ions’ motion, mechanical vibration of the magnets and the collisions themselves. Whatever the cause, more diffuse beams will produce lower luminosity and fewer collisions. So to improve luminosity, accelerator physicists at RHIC aim to eliminate, or reduce, the build-up of heat within the bunches by using electron cooling.
In 1966 Gersh Budker, of what is now the Budker Institute of Nuclear Physics (BINP) in Novosibirsk, invented electron cooling, which has been applied at numerous storage rings around the world. The idea is very intuitive: bring cold electrons into contact with the ions so that heat can flow from the warmer ions to the colder electrons. Cold electrons are produced by an electron source and are then accelerated to match precisely the speed of the ions in a straight section of the ring. Here, the two beams overlap and have a chance to exchange heat. The electrons are discarded after one pass and replaced by fresh electrons to continue the cooling process. At RHIC, which has two 3800 m rings, this straight section will be more than 100 m long. There are other differences between RHIC and previous electron-cooled rings: RHIC will be the first collider to be cooled during collisions and will be the first cooler using bunched electron beams.
To gain confidence in the calculated performance of the RHIC electron-cooler, the team at BNL has strived to develop dependable simulation techniques and benchmark them in experiments. Many institutes have helped in this challenge: BINP, JINR, Tech-X Corporation, Jefferson Laboratory, Fermilab, and the Svedberg Laboratory. The last two laboratories also helped in benchmarking experiments on their electron coolers.
One of the challenges of cooling RHIC lies with the machine’s high energy, which is around 10 times higher than any previous electron cooler (54 MeV electron energy for RHIC’s gold ions at 100 GeV per nucleon). This slows electron cooling because the cooling time is approximately proportional to the cube of the energy. The cooler therefore requires an electron beam that has a high energy and a high current. It must also cool over a long straight section, which means that a conventional DC electron accelerator cannot be used for cooling RHIC. For this reason, an ERL electron accelerator was adopted by BNL to produce electron bunches with a high charge (about 5 nC), a low emittance (under 3 μm normalized rms) and a high energy of 54 MeV. Another challenge is matching precisely – in position, speed and angular deviation – the electrons to the ions.
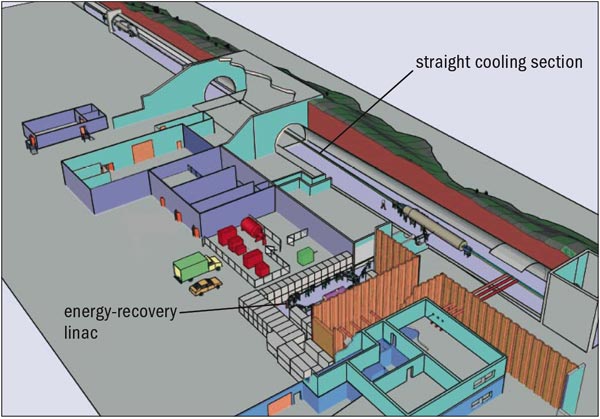
Image credit: BNL.
Figure 1 shows a possible layout of an electron cooler at RHIC. The cooling will take place in a 100 m straight section in the RHIC tunnel between two superconducting RHIC quadrupoles. The electron beam, generated by a 54 MeV superconducting RF ERL, will first travel with the beam in the anticlockwise ring and then loop back and travel with the beam in the clockwise ring. In doing so, the electron beam cools both rings.
The task of producing the necessary low-emittance and high-charge (high-brightness) electron bunches is even more difficult. The BNL team is currently working on a laser-photocathode superconducting radiofrequency source for the continuous production of a high-brightness electron beam that is capable of about 0.1 A. The design aims for a 0.5 A continuous average current. To make the ERL work without beam breakup, a superconducting accelerator cavity has been developed, which is capable of more than 3 A without beam-breakup, together with other technologies for accelerating a very high current efficiently.
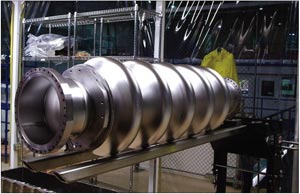
Image credit: BNL.
Following several years of intensive R&D, we are confident that, according to our calculations, these techniques will increase the luminosity at RHIC and allow more sensitive, precision studies of the substructure of matter.

Image credit: BNL.
Figure 2 shows an ERL superconducting cavity and figure 3 gives the results of a cooling simulation. The five-cell cavity, developed by the C-AD and built by local industry (Advanced Energy Systems), is the first dedicated ERL cavity to be developed. After chemistry and testing at Jefferson Laboratory, it demonstrated 20 MeV acceleration at low-power investment.
The accelerator technologies that we are developing may also have applications at BNL beyond the RHIC-II upgrade. For example, the eRHIC upgrade would add electrons from an ERL to collide with the ion beams of RHIC. Another possible application could be at future “light source” facilities, using very high brightness X-rays to study the properties of materials and biological samples.