At 11.28 p.m. on 30 June 2007, DESY’s HERA collider was shut down after almost 16 years of operation. In this article, Dieter Trines looked back at the challenge of building the first and only electron–proton collider, based on a superconducting-magnet ring.
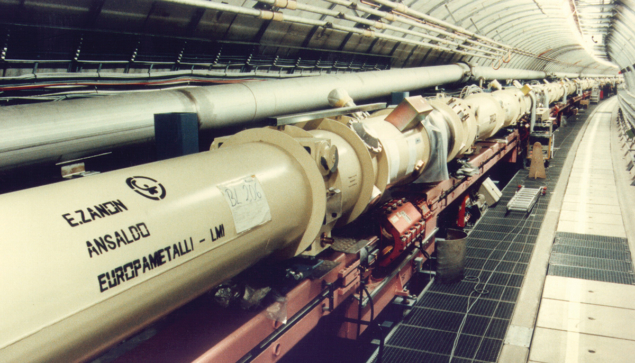
Ideas for an electron–proton collider based on storage rings first arose after the famous experimental results on deep inelastic electron–proton scattering from SLAC in 1969, which indicated a granular structure for the proton. Using two storage rings to collide electrons and protons head-on, rather than directing an electron beam at a proton target, would allow for higher centre-of-mass energies. This would in turn result in a better resolution for measurements of the internal structure of the proton. So, in the early 1970s, several laboratories – Brookhaven, CERN, DESY, Fermilab, IHEP (Moscow), Rutherford Laboratory, SLAC and TRIUMF – began to think about building an electron–proton collider.
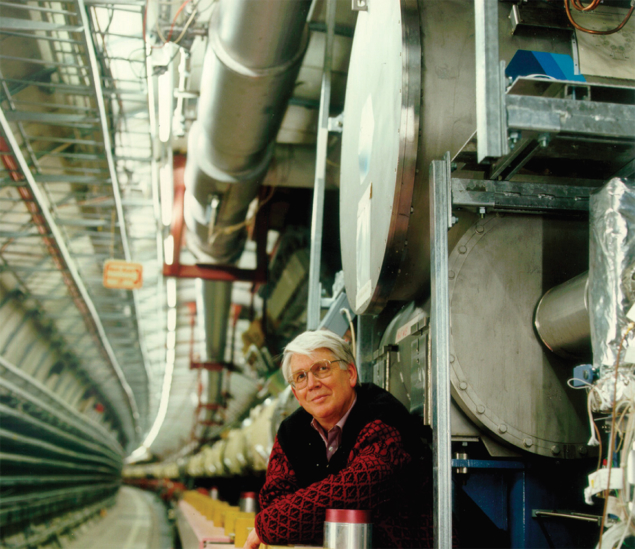
At DESY, Bjørn Wiik in particular was a major advocate for the construction of an electron–proton collider. In 1972, Horst Gerke, Helmut Wiedemann, Günter Wolf and Wiik wrote a first report in which they proposed using the existing double-storage ring DORIS for electron–proton collisions. Then, in 1981, after several workshops organized by the European Committee for Future Accelerators (ECFA), DESY submitted a proposal to the government of the Federal Republic of Germany (FRG) for the construction of a completely new machine called HERA. It was to be an electron–proton collider with a circumference of 6.3 km and it had the strong support of the European high-energy physics community and ECFA.
Early discussions on electron–proton colliders had already considered the use of superconducting magnets for the proton ring. Then, in the 1980s, this demanding technology became feasible for large systems, thanks to the courageous and pioneering work at Fermilab on superconducting magnets for the construction of the Tevatron. When it came into operation in 1983, the Tevatron was the world’s first superconducting synchrotron at high energies.
DESY had no major experience in this technology, so in 1979 Hartwig Kaiser and Siegfried Wolff were sent to work with colleagues at Fermilab and profit from their know-how. The successful dipole and quadrupole magnets developed at Fermilab naturally influenced the design of the superconducting accelerator magnets for HERA, and the first dipoles built at DESY were basically copies of the Fermilab magnets. However, with increasing experience, the physicists and engineers at DESY started to add major improvements of their own, leading to the characteristic design of the HERA magnets, which proved extremely successful over the lifetime of the accelerator. As the superconducting magnet ring was the most challenging part of HERA, this article will focus on its design in particular.
The superconducting coil is the most critical component of a superconducting magnet. Coils several metres long are fabricated with cross-sections accurate to a few hundredths of a millimetre. This demanding task was solved at Fermilab by using laminated tooling for the production and curing of the coils. These are surrounded by collars punched from stainless-steel sheets, which provide the precise coil geometry and sustain the huge magnetic forces. Only special types of steel, which do not become brittle or magnetic at cryogenic temperatures, are suitable. For the coils of the HERA dipoles, the collars are made from an aluminium alloy with high yield-strength, thus eliminating magnetic effects.
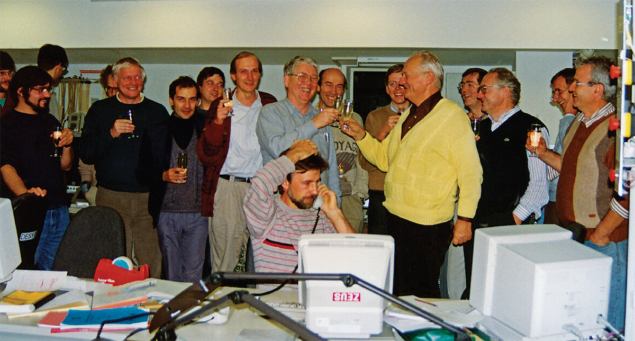
In the HERA dipoles, this collaring is reinforced by the iron yoke, which, unlike its Fermilab counterpart, is located inside the cryostat. This “cold iron” concept has several advantages. First, it leads to an additional gain of 12% in the central magnetic field, as the iron is closer to the coil. Second, the cryogenic load at 4 K is reduced as a result of the longer support rods. Finally, a passive protection scheme with parallel diodes can protect the coil against damage from the stored energy should it become normally conducting (quench). The resulting larger cold mass leads to longer cool-down and warm-up times of about five days. However, this turned out to be no drawback as there were only a few occasions during the whole lifetime of HERA, outside regular shutdowns, when the magnets had to be warmed up. Hartwig Kaiser, Karl Hubert Mess and Peter Schmüser were the main people responsible for this development.
In a superconducting magnet ring, the protection of the coils against quenches is of utmost importance and is a challenging technology in itself. It involves both the detection of a quench (by monitoring the voltage over the coils) and the installation of quench heaters to force the quenching coil to become normally conducting, thus distributing the energy deposited by the magnet current over its whole length. As many magnet coils are powered serially in long strings, the current coming from the power supply has to be bypassed around the quenching magnet and its stored energy safely dissipated in a resistive load. A switch is required to bypass the magnet. At Fermilab, this was in the form of a thyristor mounted outside the vacuum vessel, which had to be triggered in case of a quench. The current leads to the thyristor were connected to the coil at 4 K, thus adding to the cryogenic load.
For the HERA magnets, Mess applied a different idea, first considered at Brookhaven, in which a “cold” diode inside the cryostat at 4 K automatically switches the current of about 5000 A in case of a quench. This was one of the most innovative and courageous technological steps of the HERA project. First, a suitable diode had to be found. Of course, no commercially available diodes were made for such an application. Mess did eventually find one that promised to be up to the task, but only after extensive searching and testing. Then, the mechanical mounting and electrical connections of the diodes had to be devised in such a way as to guarantee their reliable operation inside the helium, where they were exposed to rapid and extreme thermal cycles. Comprehensive tests of all of the diodes were carried out to qualify them and validate the design of the mounting – an example of innovative engineering at its best.
To save costs and keep the cryogenic load at 4 K as low as possible, the various corrector magnets were connected via superconducting cables inside the 4 K helium pipe over an octant of the ring. The cables were held in a special fixture between the magnets and had to be soldered together before the 4 K helium tubes were joined by a welding sleeve. To make sure that all of the 20 or so cables were correctly connected, clever clamping devices – which supplied electrical contact to all of the wires simultaneously – were installed at two intersections. By applying voltages to the various contacts, a computerized central measuring station determined whether the cables were connected correctly in a time-effective way. There are many other cases that required ingenious ideas, such as solving the problem with persistent currents in the superconducting coils as Schmüser and his students did, but unfortunately it is impossible to cover these in a short article.
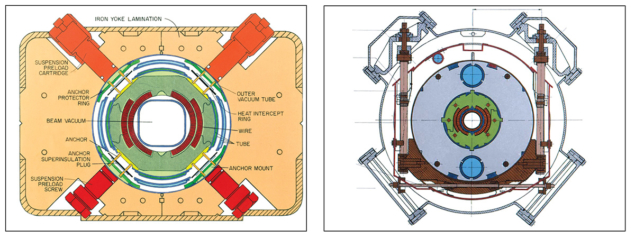
There were many systems for the superconducting magnet ring where little or no experience existed at DESY. One example is the huge cryogenic system with the cryogenic plant and the magnet cryostats, various cryogenic boxes, and transfer lines and pipes for cold and room-temperature helium, respectively. One pipe, the quench gas-collection pipe, was connected to the 4 K helium volume of the dipole magnets but separated by a special valve, named the Kautzky valve after its inventor at Fermilab. This valve opens automatically when the pressure inside the cryostat exceeds a preset value. It is sealed by a conical plastic piece inside a conical body. However, some of these valves would start to rattle during a quench, indicating that they were closing and opening in rapid succession. This effect quite often cracked the plastic cone, so the valves would no longer seal for normal operation and had to be exchanged. Despite intensive studies and tests of various materials – the high radiation level in the HERA tunnel meant that the Teflon of the original design could not be used – the problem was never solved. It did not become an operational problem thanks to the small number of quenches. This was an example where the work on HERA did not evolve from the heritage of Fermilab.
One clear evolutionary step, however, was the strong involvement of industry in the production of the superconducting magnets for HERA. For European industry in particular, HERA presented a unique opportunity: it was the first time that companies had an opportunity to gain experience in superconducting technologies and cryogenics on such a large scale. This step was beneficial for both DESY and the industrial companies, and also for later projects using superconducting-magnet technology.
Another step forward, this time in terms of financing and organizing large research projects, was the construction of HERA in collaboration with research laboratories from other countries: the so-called “HERA model”. It is to the credit of both Wiik and Volker Soergel that they brought the collaboration together with contributions from Canada, France, Israel, Italy, the Netherlands and the US, with additional manpower provided by institutes in China, Czechoslovakia, Poland, Switzerland, the UK, and USSR as well as institutes from both the FRG and the German Democratic Republic. The particularly large contribution by Italy of half of the superconducting dipoles cannot be overemphasized, and was to the great merit of Antonino Zichichi, who made this happen.
At DESY, we clearly stood on the shoulders of Fermilab’s pioneering work when realizing HERA, and the experiences and technological advancements made at HERA were valuable for later projects, such as RHIC at Brookhaven and the LHC at CERN. When DESY began the adventure of constructing the superconducting magnet ring for HERA, several people were worried that there would be problems with such a novel system and that its operation would become very difficult. Fortunately, none of the worries were substantiated and the operation of the “cold” ring essentially went without problems. I am sure that people at CERN now have similar worries concerning the LHC. I would like to express my best wishes to them, with the hope that they might be as fortunate and successful with the LHC as we were with HERA.