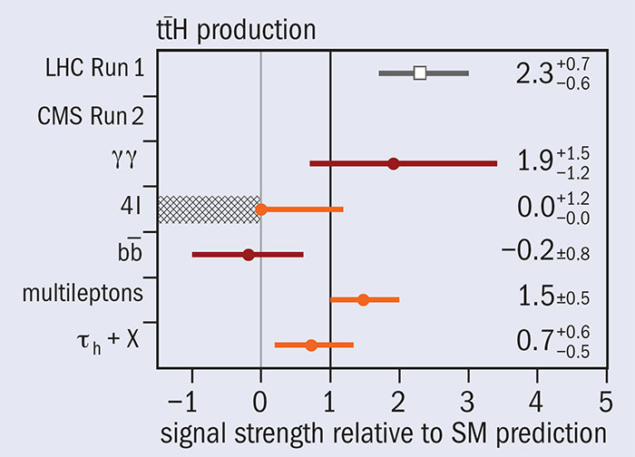
The discovery of the Higgs boson in 2012, a fundamentally new type of scalar particle, has provided the particle-physics community with a new tool with which to search for new physics beyond the Standard Model (SM). Originally discovered via its decay into two photons or four leptons, the SM Higgs boson is also predicted to interact with fermions with coupling strengths proportional to the fermion masses. The top quark, being the heaviest elementary fermion known, has the largest coupling to the Higgs boson. Precise measurements of such processes therefore provide a sensitive means to search for new physics.
The top-Higgs coupling is crucial for the production of Higgs bosons at the LHC, since the process with the largest production cross-section (gluon–gluon fusion) proceeds via a virtual top-quark loop. In this sense, Higgs production itself provides indirect evidence for the top-Higgs coupling. Direct experimental access to the top-Higgs coupling, on the other hand, comes from the study of the associated production of a Higgs boson and a top-quark pair. This production mode, while proceeding at a rate about 100 times smaller than gluon fusion, provides a highly distinctive signature in the detector, which includes leptons and/or jets from the decay of the two top quarks.
Combined ATLAS and CMS results on ttH production based on the LHC’s Run 1 data set showed an intriguing excess: the measured rate was above the SM prediction with a statistical significance corresponding to 2.3σ. With the increase of the LHC energy from 8 to 13 TeV for Run 2, the ttH production cross-section is expected to increase by a factor four – putting the ttH analyses in the crosshairs of the CMS collaboration in its search for new physics.

Compared to the first evidence for Higgs production in 2012, namely Higgs-boson decays into clean final states containing two photons or four leptons, the ttH process is much more rare, and the expected signal yields in these modes are just a few events. For this reason, searches for ttH production have been driven by the higher sensitivity achieved in Higgs decay modes with larger branching fractions, such as H → bb, H → WW, and H → ττ. The search in the H → bb final state is challenging because of the large background from the production of top-quark pairs in association with jets, and the results are currently limited by systematic and theoretical uncertainties.
A compromise between expected signal yield and background uncertainty can be obtained from final states containing leptons. Such analyses target Higgs decays to WW*, ZZ* and ττ pairs, and make use of events with two same-sign leptons or more than three light leptons produced in association with b-quark jets from top-quark decays. Multivariate techniques allow the background due to jets misidentified as leptons to be reduced, while similar algorithms provide discrimination against irreducible background from tt + W and tt + Z production. Events with reconstructed hadronic τ-lepton decays are studied separately.
The latest results of ttH searches at CMS (see figure) show that we are on the verge of measuring this crucial process with sufficient precision to confirm or disprove the previous observed excess. With a larger data set it should be possible to have clear evidence for ttH production by the end of Run 2.
Further reading
CMS Collaboration 2017 CMS-PAS-HIG-17-003.
CMS Collaboration 2017 CMS-PAS-HIG-17-004.