With top-quark production at the initial stage and the potential for multi-TeV collisions, CLIC has unique aspects beyond a Higgs factory.

The Compact Linear Collider (CLIC) is conceived in its first stage to be an 11 km-long electron–positron collider operating at a centre-of-mass energy of 380 GeV. Unlike other Higgs-factory proposals that start around 240 GeV, CLIC benefits at the initial stage not only from top-quark production, but also from two Higgs-boson production modes – Higgsstrahlung (e+e– → HZ) and WW fusion – giving extra complementary input for global interpretations of the data.
A defining feature of a linear collider is that its collision energy can be raised by extending its length. While the European strategy update recommended a circular hadron collider at the energy frontier as a long-term ambition, CLIC represents a compelling alternative were a circular machine found not to be feasible. CLIC has the potential to be extended in several stages up to 50 km and a maximum energy of 3 TeV, giving access to a wide range of physics processes (see “Multichannel” figure). Some important processes such as Higgsstrahlung production fall with energy, while others such as double-Higgs production require higher energies, and processes occurring through vector-boson fusion grow with energy. In general, the beyond-Standard-Model (BSM) sensitivity of scattering processes such as ZH, WW and two-fermion (including top-pair) production rises strongly with energy, so the higher-energy stages bring further sensitivity to potential new physics both indirectly and directly.
Lepton colliders can in general explore much closer to kinematic limits than hadron colliders
In contrast to the ILC (see ILC: beyond the Higgs), CLIC operates via a novel two-beam scheme, whereby radio-frequency power extracted from a high-current, low-energy drive beam is used to accelerate the colliding beams. Were a decision to be made to upgrade CLIC from 380 GeV to 1.5 TeV, the length of the main linacs would have to be extended to 29 km, as well as moving and adding accelerator modules. Going from an energy of 1.5 to 3 TeV, as well as further lengthening of the main linacs, a second drive-beam complex must be added. CLIC’s combination of Higgs- and top-factory running, and multi-TeV extension potential, makes it illuminating to study the physics prospects of the initial stage in parallel with those of the ultimate energy.
Higgs physics
At 380 GeV, with 1 ab–1 of integrated luminosity CLIC would produce around 160,000 Higgs bosons. This stage would enable precision determinations well beyond the HL-LHC, for example in the single-Higgs couplings to WW, ZZ, bb, and cc. Due to the known kinematic constraints in the collision environment, it also allows an absolute determination of the Higgs couplings, as opposed to the ratios accessible at the LHC. The corresponding precision on Higgs-coupling measurements is increased considerably by the enhanced statistics at 1.5 TeV, where CLIC could produce 1 million Higgs bosons with an integrated luminosity of 2.5 ab–1 as well as opening sensitivity to other processes. A linear collider like CLIC provides considerable flexibility, for example: collecting at 380 GeV 1 ab–1 in 8 years or 4 ab–1 in 13 years, as studied recently, before a possible jump to 1.5 TeV.
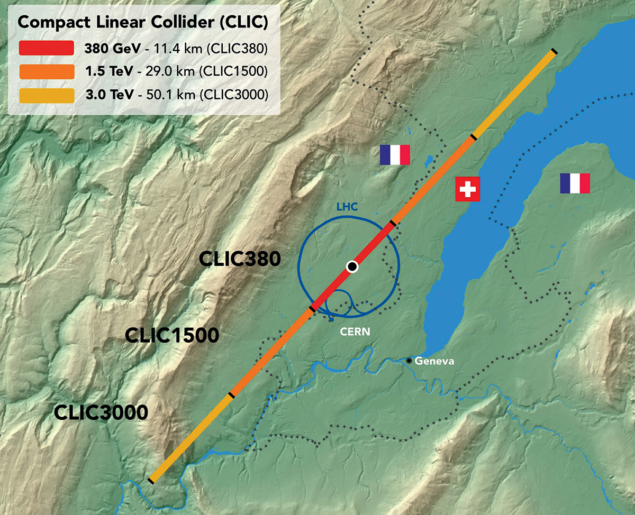
The 1.5 TeV energy stage gives access to two double-Higgs production mechanisms: double-Higgsstrahlung (e+e– → ZHH) and vector-boson fusion (e+e– → HHνeνe). Such production of Higgs-boson pairs allows the Higgs self-coupling to be probed directly. While the 1.5 TeV stage could reach a precision of –29%/+67% using a rate-only analysis, at 3 TeV an ultimate Higgs self-coupling precision of –8%/+11% is expected, also exploiting differential information. Furthermore, the ability to measure both the ZHH and HHνeνe processes allows for an unambiguous determination of the Higgs self-coupling even if it is far from its Standard Model value. Unlike indirect determinations from ZH measurements at lower Higgs-factory energies, the precision of CLIC’s direct Higgs-self-coupling measurement is largely preserved in global fits. CLIC could thus robustly verify that the Higgs self-coupling assumes the value predicted by the Standard Model, or uniquely identify the new-physics effects responsible for potential tensions with the Standard Model in Higgs observables.
Top-quark physics
CLIC is unique among the proposed electron–positron colliders in producing top-quark pairs at its initial energy stage. Electroweak couplings to third-generation fermions such as the top are particularly relevant in many BSM scenarios. Operating at the top-quark pair-production threshold of around 350 GeV would allow precise measurements of the top-quark mass and width, while cross-section and asymmetry measurements would probe the top-quark interactions. However, comprehensive exploration of top-quark couplings requires several energy stages, and spacing them widely as the CLIC baseline envisages enhances energy-dependent effects.
Electron-beam longitudinal polarisation at ±80% plays an important role in the precision programme at CLIC. Generally the polarisation significantly enhances WW-fusion processes, for example single- and double-Higgs production at higher energies; we make use of this in the baseline scenario by taking more data with left-handed electrons at the later stages. In the interpretation of Standard Model measurements, polarisation also helps to disentangle different contributions. The coupling of the top quark to the Z boson and the photon is one such example.
Indirect searches
Many observables such as cross-sections and differential distributions for WW and two-fermion production, in addition to measurements from the Higgs-boson and top-quark sectors, can be used to constrain potential new physics in the framework of effective field theory. Here, the Standard Model Lagrangian is supplemented by interaction operators of higher dimension that describe the effects of new particles. These particles could be too heavy to be produced at CLIC, but can still be probed through the effects they induce, indirectly, on CLIC observables.
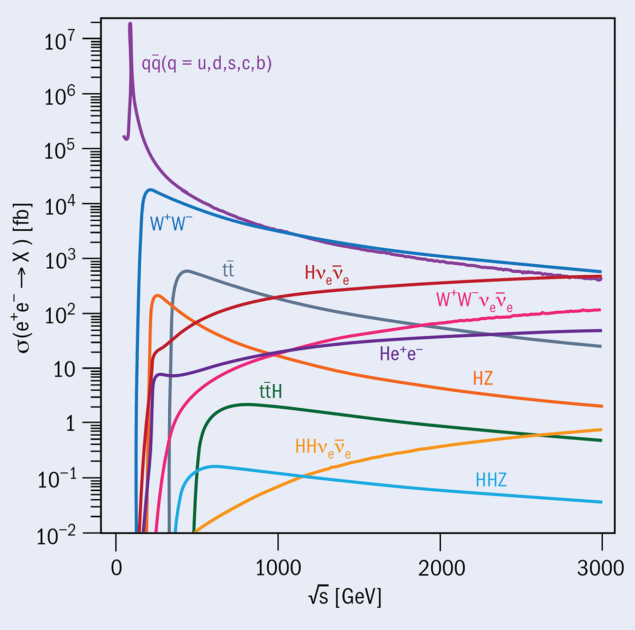
For many new-physics operators, CLIC is projected to bring an order of magnitude increase in sensitivity over the HL-LHC. The 380 GeV stage already significantly enhances our knowledge of operators relating to modifications of the Higgs couplings, as well as electroweak observables such as triple-gauge couplings. The higher-energy stages are then particularly effective in probing operators that induce corrections to Standard Model predictions which grow with energy. Sensitivity to these operators allows a wide range of new-physics scenarios to be probed without reference to particular models. Comparisons performed for the 2020 update of the European strategy for particle physics show, for example, that sensitivities derived in this way to four-fermion, or two-fermion two-boson contact interactions rise very steeply with the centre-of-mass energy of a lepton collider, allowing CLIC to probe scales up to 100 TeV and beyond.
Precision measurements of Standard Model processes can also be interpreted in the context of particular BSM models, such as the broad classes of composite Higgs and top, or extra-dimension models. At CLIC this represents strong new-physics reach. For example, a 3 TeV CLIC has sensitivity to Higgs compositeness up to a scale of around 18 TeV for all values of the compositeness sector coupling strength (see “Sensitivity” figure, left), and can reach beyond 40 TeV in particularly favourable scenarios; in all cases well beyond what the HL-LHC can exclude. At high masses, a multi-TeV lepton collider such as CLIC also provides the best possible sensitivity to search for new vector bosons such as the Y-universal Z′, which has couplings to quarks and leptons that are comparable (see figure, right).
As a further example, the very high energy of CLIC, and therefore the high propagator virtuality in two-fermion production, means that high-precision differential cross-sections could reveal deviations from Standard Model predictions owing to the presence of new particles in loops. This would allow discovery or exclusion of new states, for example dark-matter candidates, with a variety of possible quantum numbers and masses in the range of several TeV.
Direct searches
Direct searches for new physics at CLIC benefit from the relatively clean collision environment and from triggerless detector readout, both of which allow searches for elusive signatures that are difficult at a hadron collider. Mono-photon final states are an example of such a signature. In simplified dark-matter models containing a dark-matter particle and a mediator, dark-matter particles can be pair-produced in association with a photon, which is observed in the detector. In the case of a scalar mediator, lepton colliders are particularly sensitive and CLIC’s reach for the mediator can exceed its centre-of-mass energy significantly. In the case where the couplings to electrons and quarks are different, e+e– and proton colliders provide complementary sensitivities.
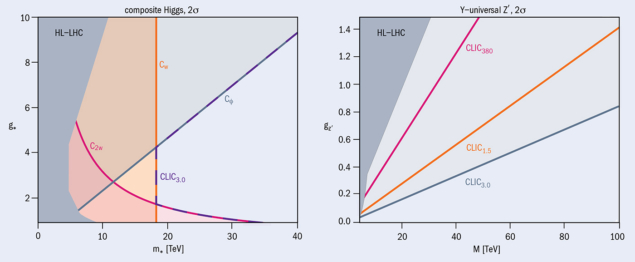
Lepton colliders can in general explore much closer to kinematic limits than hadron colliders, and this was recently verified in several examples of pair production, including simplified supersymmetric models and doubly charged Higgs production. Supersymmetric models where the higgsino multiplet is decoupled from all other supersymmetric states can lead to charginos decaying to slightly lighter neutralinos and leaving a “disappearing track stub” signature in the detector. CLIC at 3 TeV would be sensitive to such a higgsino to masses beyond 1.1 TeV, which is what would be required for the higgsino to account for the dark-matter relic mass density.
All the above approaches can be combined to illuminate the electroweak phase transition in the early universe. Models of electroweak baryogenesis can contain new scalar particles to facilitate a strong first-order phase transition, during which the electroweak symmetry is broken. Such scalar singlet extensions of the Higgs sector can be searched for directly; and indirectly from a universal scaling of all Higgs couplings.
Having both the precision capacity of a lepton collider and also the high-energy reach of multi-TeV collisions, CLIC has strong potential beyond a Higgs factory as a discovery machine. Over the next five years CERN will maintain a level of R&D in key CLIC technologies, which are also being adapted for medical applications, such that the project could be realised in a timely way after the HL-LHC if the international community decides to take this route.
Further reading
J de Blas et al. 2018 CERN Yellow Reports CERN-2018-009-M.
P Burrows et al. 2018 CERN Yellow Reports CERN-2018-005-M.
A Robson et al. 2020 arXiv:2001.05278.