Fifty years ago, neutrino beams traversing the Gargamelle bubble chamber revealed the existence of the neutral current and put the electroweak Standard Model on solid ground. Sanje Fenkart describes Gargamelle’s feat and the lasting impact on CERN’s neutrino programme.
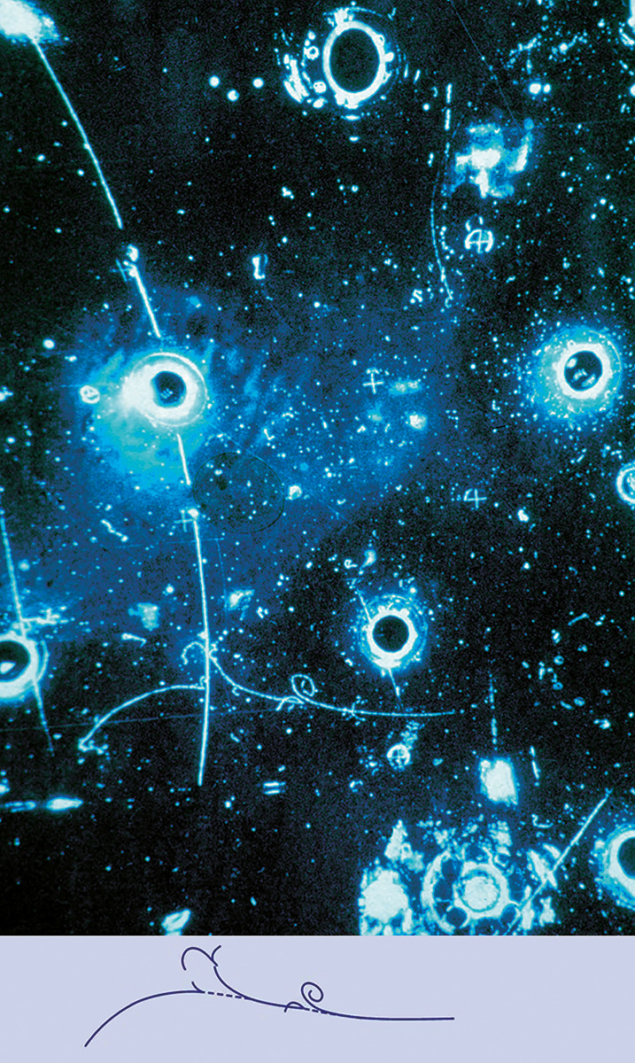
The neutrino had barely been known for two years when CERN’s illustrious neutrino programme got under way. As early as 1958, the 600 MeV Synchrocyclotron enabled the first observation of the decay of a charged pion into an electron and a neutrino – a key piece in the puzzle of weak interactions. Dedicated neutrino-beam experiments began a couple of years later when the Proton Synchrotron (PS) entered operation, rivalled by activities at Brookhaven’s higher-energy Alternating Gradient Synchrotron in the US. Producing the neutrino beam was relatively straightforward: make a proton beam from the PS hit an internal target to produce pions and kaons, let them fly some distance during which they can produce neutrinos when they decay, then use an iron shielding to filter the remaining hadrons, such that only neutrinos and muons remain. Ensuring that a new generation of particle detectors would enable the study of neutrino-beam interactions proved a tougher challenge.
CERN began with two small, 1 m-long heavy-liquid bubble chambers that used proton beams which struck an internal target inside the PS, hoping to see at least one neutrino event per day. It was nowhere near that. Unfortunately the target configuration had made the beams about 10 times less intense than expected, and in 1961 CERN’s nascent neutrino programme came to a halt. “It was a big disappointment,” recalls Don Cundy, who was a young scientist at CERN at the time. “Then, several months later, Brookhaven did the same experiment but this time they put the target in the right place, and they discovered that there were two neutrinos – the muon neutrino (νµ) and the electron neutrino (νe) – a great discovery for which Lederman, Schwartz and Steinberger received the Nobel prize some 25 years later.”
Despite this setback, CERN Director-General Victor Weisskopf, along with his Director of Research Gilberto Bernardini and the CERN team, decided to embark on an even more ambitious setup. Employing Simon van der Meer’s recently proposed “magnetic horn” – a high-current, pulsed focusing device placed around the target – and placing the target in an external beam pipe increased the neutrino flux by about two orders of magnitude. In 1963 this opened a new series of neutrino experiments at CERN. They began with a heavy-liquid bubble chamber containing around 500 kg of freon and a spark-chamber detector weighing several tonnes, for which first results were presented at a conference in Siena that year. The bubble-chamber results were particularly impressive, recalls Cundy: “Even though the number of events was of the order of a few hundred, you could do a lot of physics: measure the elastic form factor of the nucleon, single pion production, the total cross section, search for intermediate weak bosons and give limits on neutral-current processes.” It was at that conference that André Lagarrigue of Orsay urged that bubble chambers were the way forward for neutrino physics, and proposed to build the biggest chamber possible: Gargamelle, named after a giantess from a fictional renaissance story.
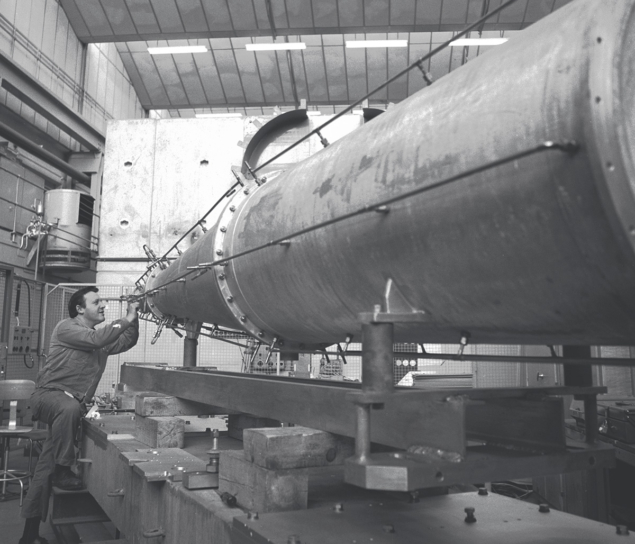
Construction in France of the much larger Gargamelle chamber, 4.8 m long and containing 18 tonnes of freon, was quick, and by the end of 1970 the detector was receiving a beam of muon neutrinos from the PS. The Gargamelle collaboration consisted of researchers from seven European institutes: Aachen, Brussels, CERN, École Polytechnique Paris, Milan, LAL Orsay and University College London. In 1969 the collaboration had made a list of physics priorities. Following the results of CERN’s Heavy Liquid Bubble Chamber, which set new limits on neutrino-electron scattering and single-pion neutral-current (NC) processes, the search for actual NC events made it onto the list. However, it only placed eighth out of 10 science goals. That is quite understandable, comments Cundy: “People thought that the most sensitive way to look for NCs was the decay of a K0 meson into two muons or two electrons but that had a very low branching ratio, so if NCs existed it would be at a very small level. The first thing on the list for Gargamelle was in fact looking at the structure of the nucleon, to measure the total cross section and to investigate the quark model.”
Setting priorities
After the discovery of the neutrino in 1956 by Reines and Cowan (CERN Courier July/August 2016 p17), the weak interaction became a focus of nuclear research. The unification of the electromagnetic and weak interactions by Salam, Glashow and Weinberg a decade later motivated experiments to look for the electroweak carriers: the W boson, which mediates charged-current interactions, and the Z boson associated with neutral currents. While the former were known to exist by means of β decay, the latter were barely thought of. Neutral currents started to become interesting in 1971, after Martinus Veltman and Gerard ’t Hooft proved the renormalisability of the electroweak theory.
More than 60 years after first putting the neutrino to work, CERN’s neutrino programme continues to evolve
By that time, Gargamelle was running at full speed. Analysing the photographs that were taken every time the PS was pulsed to look for interesting tracks were CERN personnel (at the time often referred to as “scanning girls”) who essentially performed the role of a modern level-1 trigger. Interactions were divided into different classes depending on the number of particles involved (muons, hadrons, electron–positron pairs, even one or more isolated protons as well as isolated electrons and positrons). The leptonic NC process (νµ + e– → νµ + e–) would give an event that consisted of a single energetic electron. Since the background was very low, it would be the smoking gun for NCs. However, the cross-section was also very low, with only one to nine events expected from the electroweak calculations. The energetic hadronic NC event (νµ + N → νµ + X, with the respective process involving antiparticles if the reaction was triggered by an antineutrino beam) would consist only of several hadrons, in fact just like events produced by incoming high-energy neutrons.
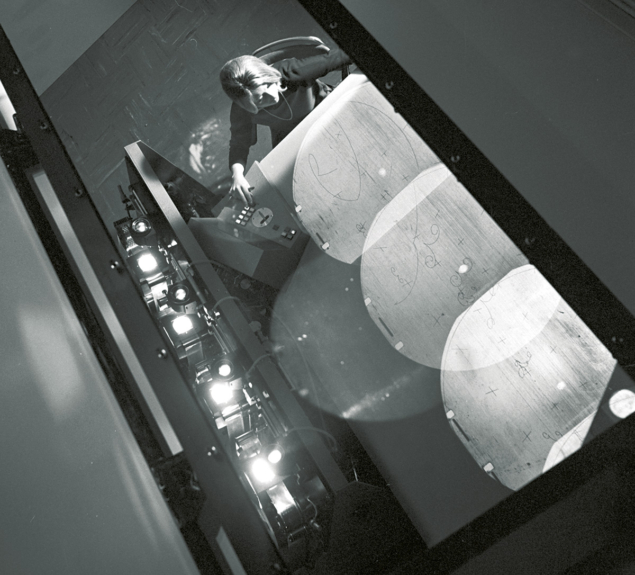
“When the first leptonic event was found in December 1972 we were convinced that NCs existed,” says Gargamelle member Donatella Cavalli from the University of Milan. “It was just one event but with very low background, so a lot of effort was put into the search for hadronic NC events and in the full understanding of the background. I was the youngest in my group and I remember spending the evenings with my colleagues scanning the films on special projectors, which allowed us to observe the eight views of the chamber. I proudly remember my travels to Paris, London and Brussels, taking the photographs of the candidate events found in Milan to be checked with colleagues from other groups.”
At a CERN seminar on 19 July 1973, Paul Musset, who was one of the principal investigators, presented Gargamelle’s evidence for NCs based on both the leptonic and hadronic analyses. Results from the former had been published in a short paper received by Physics Letters two weeks earlier, while the paper on the hadronic events, which reported on the actual observation and hence confirmation of neutral currents, was received on 23 July. In August 1973 Gerald Myatt of University College London, now at the University of Oxford, presented the results at the Electron-Photon conference. The papers were published in the same issue of the journal on 3 September. Yet many physicists doubted them. “It was generally believed that Gargamelle made a mistake,” says Myatt. “There was only one event, a tiny track really, and very low background. Still, it was not seen as conclusive evidence.” Among the critical voices were T D Lee, who was utterly unimpressed, and Jack Steinberger, who went as far as to bet half his wine cellar that the Gargamelle result would be wrong.
The difficulty was to demonstrate that the hadronic NC signal was not due to background from neutral hadrons. “A lot of work and many different checks were done, from calculations to a full Monte Carlo simulation to a comparison between spatial distributions of charged- and neutral-current events,” explains Cavalli. “We were really happy when we published the first results from hadronic and leptonic NCs after all background checks, because we were confident in our results.” Initially the Gargamelle results were confirmed by the independent HPWF (Harvard–Pennsylvania–Wisconsin–Fermilab) experiment at Fermilab. Unfortunately, a problem with the HPWF setup led to their paper being rewritten, and a new analysis presented in November 1973 showed no sign of NCs. It was not until the following year that the modified HPWF apparatus and other experiments confirmed Gargamelle’s findings.
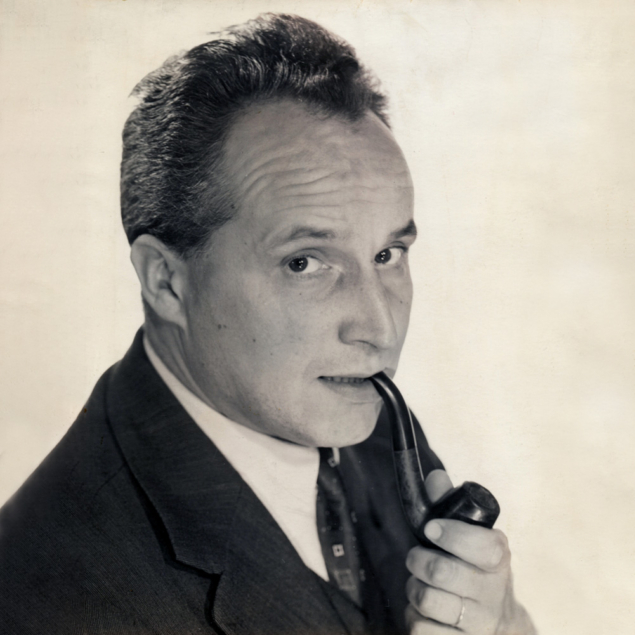
Additionally, the collaboration managed to tick off number two on its list of physics priorities: deep-inelastic scattering and scaling. Confirming earlier results from SLAC which showed that the proton is made of point-like constituents, Gargamelle data were crucial in proving that these constituents (quarks) have charges of +2/3 and –1/3. For neutral currents, the icing on the cake came 10 years after Gargamelle’s discovery with the direct discovery of the Z (and W) bosons at the SppS collider in 1983. The next milestone for CERN in understanding weak interactions came in 1990 with the precise measurement of the decay width of the Z boson at LEP, which showed that there are three and no more light neutrinos.
Legacy of a giantess
In 1977 Gargamelle was moved from the PS to the newly installed Super Proton Synchrotron (SPS). The following year, however, metal fatigue caused the chamber to crack and the experiment was decommissioned. Some of the collaboration members – including Cundy and Myatt – went to work on the nearby Big European Bubble Chamber. Also hooked up to the SPS for neutrino studies at that time were CDHS (CERN–Dortmund–Heidelberg–Saclay, officially denoted WA1) led by Steinberger, and Klaus Winter’s CHARM experiment. Operating for eight years, these large detectors collected millions of events that enabled precision studies on the structure of the charged and neutral currents as well as the structure of nucleons and the first evidence for QCD via scaling violations.
The third type
The completion of the CHARM programme in 1991 marked the halt of neutrino operations at CERN for the first time in almost 30 years. But not for long. Experimental activities restarted with the search for neutrino oscillations, driven by the idea that neutrinos were an important component of dark matter in the universe. Consequently, two similarly styled short-baseline neutrino-beam experiments – CHORUS and NOMAD – were built. These next-generation detectors, which took data from 1994 to 1998 and from 1995 to 1998, respectively, joined others around the world to look for interactions of the third neutrino type, the ντ, and to search for neutrino oscillations, i.e. the change in neutrino flavour as they propagate, which was proposed in the 1950s and confirmed in 1998 by the SNO and Super-Kamiokande experiments in Canada and Japan. In 2000 the DONUT experiment at Fermilab reported the first direct evidence for ντ interactions.
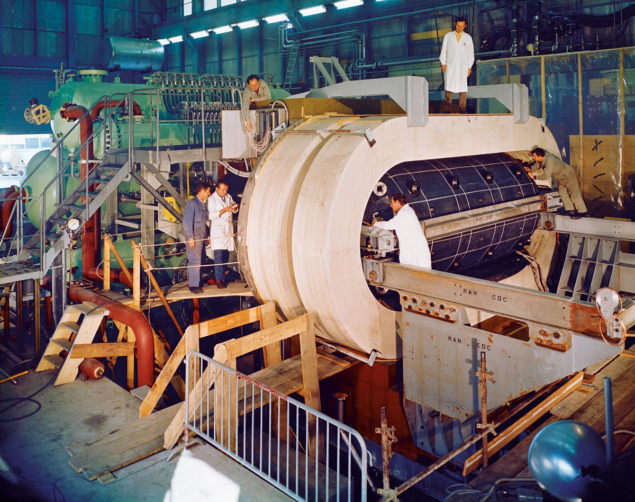
CERN’s neutrino programme entered a hiatus until July 2006, when the SPS began firing an intense beam of muon neutrinos 732 km through Earth to two huge detectors – ICARUS and OPERA – located underground at Gran Sasso National Laboratory in Italy. Designed to make precision measurements of neutrino oscillations, the CERN Neutrinos to Gran Sasso (CNGS) programme observed the oscillation of muon neutrinos into tau neutrinos and was completed in 2012.
As the CERN neutrino-beam programme was wound down, a brand-new initiative to support fundamental neutrino research began. “The initial idea for a ‘neutrino platform’ at CERN was to do a short-baseline neutrino experiment involving ICARUS to check the LSND anomaly, and another to test prototypes for “LBNO”, which would have been a European long-baseline neutrino oscillation experiment sending beams from CERN to Phyäsalmi in Finland to investigate the oscillation,” says Dario Autiero, who has been involved in CERN’s neutrino programme since the beginning of the 1980s. “The former was later decided to take place at Fermilab, while for the latter the European and US visions for long-baseline experiments found a consensus for what is now DUNE (the Deep Underground Neutrino Experiment) in the US.”
A unique facility
Officially launched in 2013 in scope of the update to the European strategy for particle physics, the CERN Neutrino Platform serves as a unique R&D facility for next-generation long-baseline neutrino experiments. Its most prominent project is the design, construction and testing of prototype detectors for DUNE, which will see a neutrino beam from Fermilab sent 1300 km to the SURF laboratory in Dakota. One of the Neutrino Platform’s early successes was the refurbishment of the ICARUS detector, which is now taking data at Fermilab’s short-baseline neutrino programme. The platform is also developing key technologies for the near detector for the Tokai-to-Kamioka (T2K) neutrino facility in Japan (see p10), and has a dedicated theory working group aimed at strengthening the connections between CERN and the worldwide neutrino community. Independently, the NA61 experiment at the SPS is contributing to a better understanding of neutrino–nucleon cross sections for DUNE and T2K data.
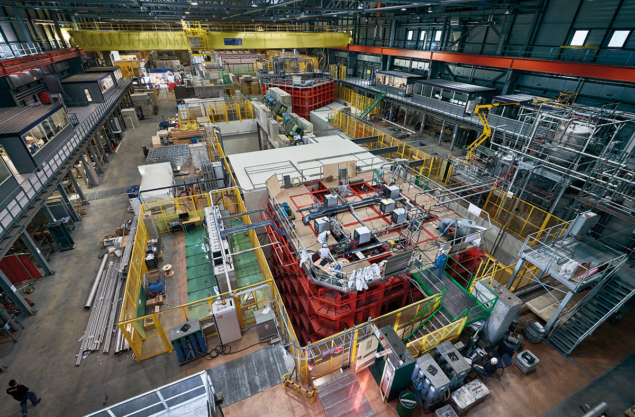
More than 60 years after first putting the neutrino to work, CERN’s neutrino programme continues to evolve. In April 2023 a new experiment at the LHC called FASER made the first observation of neutrinos produced at a collider. Together with another new experiment, SND@LHC, FASER will enable the study of neutrinos in a new energy range and compare the production rate of all three types of neutrinos to further test the Standard Model.
As for Gargamelle, today it lies next to BEBC and other retired colleagues in the garden of Square van Hove behind CERN’s main entrance. Not many can still retell the story of the discovery of neutral currents, but those who can share the story with delight “It was very tiny that first track from the electron, one in hundreds of thousands of pictures,” says Myatt. “Yet it justified André Lagarrigue’s vision of the large heavy-liquid bubble chamber as an ideal detector of neutrinos, combining large mass with a very finely detailed picture of the interaction. There can be no doubt that it was these features that enabled Gargamelle to make one of the most significant discoveries in the history of CERN.”