After 40 years of research, physicists met at the First International Workshop on the Radio Detection of High Energy Particles to discuss the detection of high-energy cosmic rays and neutrinos using radio waves. Peter Gorham and David Saltzberg report.
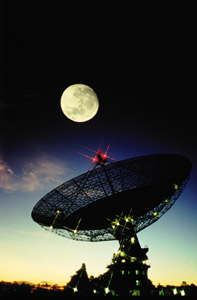
The highest-energy particles ever observed are also the rarest of all observed cosmic rays – only a few per square kilometre per century reach the Earth. Understanding the origin of these particles, with energies measured up to 300 x 1018 eV (more than 1 million times as much energy as accelerators on Earth) is at the heart of questions posed by particle astrophysicists: Where and how are these particles produced and accelerated? How can they reach the Earth without losing their energy? Are they indicative of as yet unknown physics, such as extremely heavy relics of the Big Bang? In response, physicists and astrophysicists have tried to collect as many of these elusive events as possible. They have proposed and built the largest particle detectors ever considered, with volumes measured in cubic kilometres.
Coherence from cascades
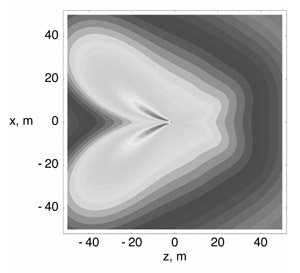
Since the early 1960s, astrophysicists have recognized that a special property of radio emission from particle cascades, known as coherence, could be the basis of the largest particle detectors. For detectors of visible light, the intensity of light increases in direct proportion to the energy deposited by a particle showering in a material. However, for radio waves having wavelengths several times as large as the size of the shower, each particle produces electromagnetic radiation in phase, coherently, so that the total electric field increases in direct proportion to the shower energy. The resulting quadratic increase of radio emission with particle energy means that radio emission from an ultrahigh-energy cascade will dominate all other forms of secondary radiation. It is this that drew more than 50 physicists and astrophysicists from around the world in November 2000 to the University of California, Los Angeles, to the the First International Workshop on the Radio Detection of High Energy Particles (RADHEP-2000).
The history of theoretical ideas was reviewed by Boris Bolotovskii (LPI, Moscow) and Jon Rosner (Chicago). In the 1960s several mechanisms were identified that would cause the particles to radiate. The Armenian-Russian theorist Gurgen Askaryan from LPI realized that Compton scattering and positron annihilation by the shower particles in matter would result in a 15-25% excess of electrons over positrons that could emit radio Cherenkov radiation. Kahn and Lerche pointed out that the splitting of the electron and positron trajectories in the Earth’s magnetic field would cause them to radiate like a relativistic dipole.
Giant spark chamber
Robert Wilson, later the founding director of Fermilab, even considered the possibility that cosmic rays could discharge the atmosphere’s electric field gradient like a giant spark chamber. In air, the radiation will be coherent for frequencies of up to about 100 MHz. In dense materials, where the shower is more compact, coherence may be seen up to about 10 GHz. At the workshop banquet, Bolotovskii gave a memorable after-dinner personal reminiscence of Askaryan, whose sharp mind led to many ground-breaking physics ideas as well as to personal and professional struggles. Karo Ispirian (Yerevan, Armenia) translated and recited several of Askaryan’s poems.
Trevor Weekes (Harvard-Smithsonian) recounted the story of the first successful radio detection of cosmic rays in 1964 – the result of his PhD work at Jodrell Bank with Neil Porter in an experiment supervised by John Jelley, one of the pioneers of Cherenkov detection techniques. Weekes showed pulses that were probably the first radio emissions detected from extensive air showers induced by cosmic rays with energies of more than 1016 eV. The data-acquisition system consisted of a camera mounted on an oscilloscope triggered by a small array of Geiger counters. The camera recorded the scope trace of the summed voltage output of a large field of dipole antennas. Weekes noted that the number of papers presented at cosmic-ray conferences on radio signals dropped precipitously in the mid-1970s, leading A A Watson to remark: “It appears that experimental work on radio signals has been terminated everywhere.”
Yet from 1979 to 1992, the period when few groups were working with radio techniques, the Gauhati University Cosmic-Ray Group collected a large sample of atmospheric events in India. The latest results and implications for production models were transmitted to the workshop in absentia by Kalpana Sinha (Assam Institute, India). Rosner summarized the team’s work as well as from others in Japan and the former Soviet Union in a variety of measurements ranging from 2 to 200 MHz. Rosner also described his own air-shower work at the Dugway Proving Grounds in Utah.
Augmenting Auger array
Using a simple antenna and recording system, they are approaching the sensitivity to electric fields in the 20-250 MHz range necessary to detect coincidences. This work is proceeding with an eye towards augmenting the giant Pierre Auger array under construction in Argentina with a complementary radio detector. Rosner’s talk will probably be a useful resource for future students of the field, because he has collected a large number of useful scaling laws and common experimental details in one place.
Upward-going showers in a large volume of solid material is one of the classic signatures that is sought to identify neutrino-induced events, which could give birth to high-energy neutrino astronomy. Steve Barwick (Irvine) and Francis Halzen (Wisconsin) reported on current and future experiments – Amanda and Icecube – which search for optical Cherenkov radiation from the showers produced by such events.
Radio searches for upward-going events began in earnest in the 1980s when various groups began to bury dipoles in deep Antarctic ice, which is so pure that it has been measured to be transparent to radio and microwaves for hundreds, even thousands, of metres. Dave Besson (Kansas) reported on the status of the largest and longest running of these, the Radio Ice Cherenkov Experiment (RICE). This collaboration has submerged radio dipoles at the South Pole on the strings used by the Amanda experiment for placing its phototubes 2 km below the surface. It placed a limit on the neutrino flux, although there are a few intriguing events and more data to be analysed.
Masami Chiba (Tokyo Metropolitan) discussed his measurements of nearly lossless propagation in another ultrapure material – salt. He described how natural geological salt formations may be of sufficient purity and size to provide a complementary material to Antarctic ice with more than twice the density.
Askaryan was the first to note that the outer few metres of the Moon’s surface, known as the regolith, would be a sufficiently transparent medium for detecting microwaves from the charge excess in particle showers. The radio transparency of the regolith has since been confirmed by the Apollo missions.
In the late 1980s Igor Zheleznykh (INR, Moscow) and Rustam Dagkesamanskii (LPI, Puschino), who both participated in RADHEP, predicted that radiotelescopes on Earth would be sensitive to such microwave pulses and might discover a flux of cosmic neutrinos with energies of more than 1019 eV. Tim Hankins (New Mexico Tech) reported on the first such search in 1996 using the 64 m diameter Parkes radiotelescope in Australia. Peter Gorham (Jet Propulsion Lab) reported on a current search using two large and physically separated radiotelescopes in coincidence at NASA’s Deep Space Network in Goldstone, California. Requiring coincident microwave pulses at both antennas all but eliminates triggers due to terrestrial radio interference. (The day after the workshop, many of the participants joined an excursion to the Mojave Desert, where NASA sponsored a tour of the Deep Space Network radio antennas.) Dagkesamanskii described his plans for a search using the Kalyazin and Bear-Lake Radio Telescopes in Russia.
Particle theorists who dared to predict the electric field intensities from particles showering in solids were faced with developing an interface between large shower Monte Carlo programs and Maxwell’s equations.
Modest controversy
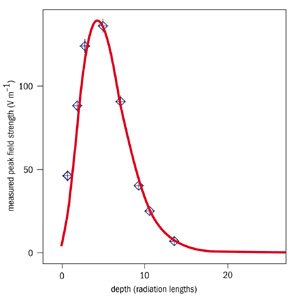
Enrique Zas (Santiago de Compostela, Spain) summarized the pioneering work by Halzen, Zas, Stanev and Alvarez-Muñiz with detailed particle showers and models for radiation that improved Askaryan’s early work. Soeb Razzaque (Kansas) described his new work using the modern GEANT Monte Carlo package. Some disagreement with the other simulations point to a modest controversy. Roman Buniy (Kansas) warned all concerned to be careful with lengthscales, such as the Fresnel versus Fraunhofer limits. Buniy showed some beautiful graphical visualizations from his calculations of field intensities and phases that show the “richness of structure” imposed by the different lengthscales. Jaime Alvarez-Muñiz (Bartol), with a major effort in three dimensional geometry, applied some of the theoretical results to the lunar experiments, describing how parameters could be varied to optimize the detection of neutrinos versus other high-energy particles.