With its mix of plenary, parallel and poster sessions, IPAC’14 put the many facets of accelerator studies on show.
Résumé
Les accélérateurs à l’honneur à Dresde
La Conférence internationale sur les accélérateurs de particules (IPAC), associant sessions plénières, sessions parallèles et affichages, est la grande rencontre annuelle sur l’actualité des accélérateurs de particules. Il y est question aussi bien de l’expérience observée avec des machines opérationnelles que des études portant sur des concepts innovants. Cette année, IPAC’14 a eu lieu à Dresde, en juin, et a rassemblé plus de 1200 participants. Il y a été question de très petits et de très grands accélérateurs, à des énergies très faibles ou très élevées ; des idées ont été échangées sur les projets futurs visant à explorer les frontières de l’énergie, de l’intensité et de la brillance dans les décennies à venir. Les applications des accélérateurs et les interactions avec l’industrie ont également figuré en bonne place au programme.
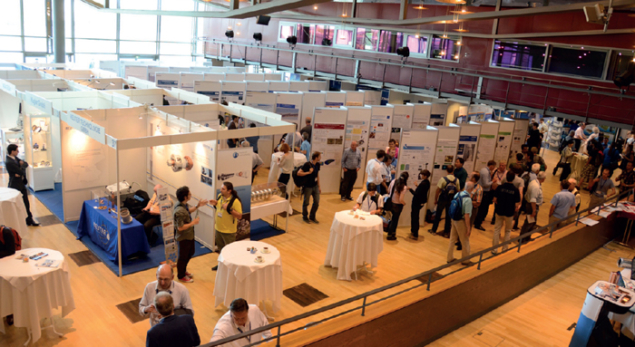
Image credit: T Pritchard.
Research in the field of accelerators ranges from investigations into the underlying physics, to R&D into new materials and methods – a span that is matched by the breadth of interest in institutes and laboratories around the world. The International Particle Accelerator Conference (IPAC) provides an annual showcase for worldwide developments in particle accelerators, from recent experience with operational machines to studies for new and innovative concepts. This year the conference, which rotates between Europe, America and Asia, took place in Dresden – the Florence of the Elbe – on 15–20 June, attracting more than 1200 participants (see box).
Topics at IPAC’14 ranged from the smallest to the largest accelerators, from the lowest to the highest energies and encompassed ideas for future projects to explore frontiers in energy, intensity and brightness in the decades to come. This report selects a few highlights, with a slant towards the use of accelerators in particle physics.
Three years ago, when IPAC was last in Europe, CERN’s LHC had a starring role as the world’s high-energy accelerator (CERN Courier December 2011 p15). Now, as the teams begin to reawaken the LHC after its first long shutdown, interest is shifting towards pushing the high-energy frontier even further. The opening talk of the conference set the bar high, with a review of the challenges for big circular colliders, in particular the Future Circular Collider (FCC) design study (CERN Courier April 2014 p16). A tunnel with a circumference of 100 km equipped with 16-T magnets – about twice the field strength of the current LHC dipoles – would allow proton–proton collisions at 100 TeV in the centre of mass. An intermediate step could be a high-luminosity circular electron–positron collider operating at a centre-of-mass energy of up to 350 GeV or higher. A high-luminosity lepton–hadron collider using the same infrastructure would be another possibility (CERN Courier June 2014 p33). A tentative timeline for such a project would see physics starting around 2035, taking the field comfortably into the second half of the century. A study for a similar but smaller electron–positron collider – the CepC – is under way in China. With a circumference of 54 km and a beam energy of up to 120 GeV, it would be associated with a 30–50 TeV proton collider – the SppC.
Such schemes present many challenges, not least in the design of high-field magnets. The High Luminosity LHC project is already driving R&D on magnets based on a niobium-tin (Nb3Sn) superconductor, which can sustain higher maximum fields than the standard niobium-titanium (Nb-Ti) compound. Collaborative work between laboratories in the US and CERN has made good progress on models and prototypes, both for interaction-region quadrupoles with a field gradient of 140 T/m and for dipoles with a nominal field of around 11 T. High-temperature superconducting materials offer the promise of reaching higher magnetic fields, but the challenge is to produce suitable cables for the magnetic-coil windings. In the case of Nb-Ti, the solution was the Rutherford cable structure. The cuprate superconductors BSCCO 2223 and REBCO are commercially available now as tape in lengths of up to a kilometre, and fields above 30 T have been achieved in solenoids. There has also been recent progress in the development of BSCCO 2212 round wire, which has been used to make Rutherford cables. By combining inserts of a high-temperature superconductor within outer windings of Nb3Sn and Nb-Ti, magnets with fields of 25–30 T could be possible.
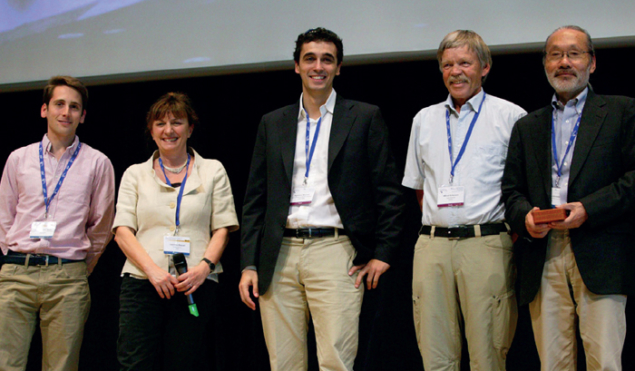
Image credit: IPAC’14.
At the opposite extreme from projects such as the FCC, the Extra Low Energy Antiproton ring (ELENA) at CERN will turn the concept behind accelerators on its head by decelerating antiprotons. The 30-m-circumference synchrotron will reduce the energy of beam from the Antiproton Decelerator (AD) from 5.3 MeV to 100 keV. The challenge here is to reduce the emittance in all three planes to allow a substantial increase in the antiproton capture efficiencies in the experiments. Design and construction are well under way, with a view to the first operation for physics in 2017.
The highest-energy accelerators for particle physics are few in number, but projects at the high-intensity frontier are increasingly required for many types of science. Here the figure of merit concerns beam power rather than energy. For neutron sources, beam power is already reaching 1 MW, while for heavy ions the Facility for Rare Isotope Beams under construction at Michigan State University aims to achieve 400 kW – two orders of magnitude greater than existing comparable facilities. The key technology in all cases is the use of superconducting radiofrequency (SRF) cavities. High powers also imply fast protection systems to prevent damage from beam loss, as well as innovative ideas for the particle sources, and there are also space-charge effects to overcome.
In particle physics, Fermilab aims to double the beam power of the Main Injector by using the Recycler to slip-stack protons during the ramp to reach 700 kW at 120 GeV. With 460 kW expected by the end of summer, the programme is on course to reach its target once RF upgrades to the Booster are complete in 2015 (CERN Courier December 2013 p24). At the Japan Proton Accelerator Complex, the plan is to reach a record intensity for a proton accelerator in the Rapid Cycling Synchrotron (RCS). Last year, the linac was upgraded from 181 MeV to the design value of 400 MeV. This allowed the RCS to demonstrate operation with beam power up to 550 kW, with low beam losses (< 0.5%) earlier this year. Operation at 1 MW is planned after the front end of the linac is replaced this summer.
High intensities, as with high energies, come at a price, and the design options have to balance the cost against technical risk. The European Spallation Source is designed as an intense source of neutrons provided by 5 MW of average proton beam power on the spallation target, with a peak of 125 MW for the study of rare processes (CERN Courier June 2014 p21). The cost, as for any high-intensity hadron linac, is driven by the RF system mainly. Based on long pulses, the ESS does not require a compressor ring and can deliver a given peak current at any beam energy. A review in 2013 led to an elegant solution centred on a reduction in energy and a corresponding increase in the gradient and peak current to keep costs down, while increasing the technical risk to some extent. However, the risk has been mitigated by reserving space to allow the installation of additional cryo-modules.
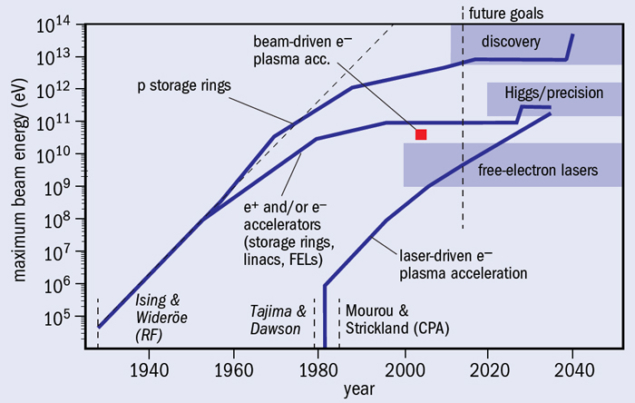
Image credit: R Assmann.
While the ESS will provide an intense source of neutrons for a variety of science, other facilities – the light sources – generate beams of X-rays or ultraviolet light. Synchrotron-radiation light sources, which began to emerge in the 1970s, are now being joined increasingly by linac-based free-electron lasers (FELs) to provide beams for studies ranging from materials science to biology. A large number of facilities have emerged during the past couple of decades in countries that are often quite small, forming an impressive worldwide community. The potential of existing facilities continues to be maximized through upgrades based on new technical innovations, while new facilities are being designed to push the limits even further.
Fourth-generation light sources aim at short time structures and ultra-high brightness by reducing the beam emittance down to the so-called diffraction limit. For storage rings this presents challenges in the design of ultra-low-emittance lattices. Machines are already being built with emittances of the order of 100 pm-rad – for example, MAX-IV in Lund, which is the first to use a highly technical multi-bend achromat lattice. Studies are also looking towards sub-10-pm-rad emittances for the future, although the solutions are likely to be costly.
The Linac Coherent Light Source (LCLS) at SLAC – a FEL making use of SLAC’s famous linac – was the first hard X-ray FEL with a peak X-ray brightness 10 orders of magnitude higher than that of the best synchrotron radiation source (CERN Courier December 2010 p17). Following the recommendation of a subcommittee of the US Department of Energy for “a new light source with revolutionary capabilities”, the project for the upgrade to LCLS-II saw a radical change in August 2013. The new plan is to build a 4-GeV continuous-wave superconducting linac in the first kilometre of the existing tunnel, based on superconducting RF cavities with a nitrogen-doping surface layer to enable the required, unprecedented performance. A new undulator will receive electrons from either the new linac (to provide 1–5 keV photons) or the existing copper linac (to provide 1–25 keV X-rays). LCLS-II is expected to deliver its first light in September 2019.
A more specialized area for the application of accelerator technology is in cancer therapy, where the requirements for the beam – in terms of emittance, intensity and stability, for example – are different from those in a nuclear-physics laboratory. Size, weight and price are also important, stimulating the application of new developments in superconductivity and novel accelerator types. In addition, the movement of the body as a result of breathing, motion of the gut and changes in the patient’s position provide challenges in beam delivery and control. Coupling the latest imaging technologies with advanced computer-modelling methods can provide a way to tell a therapist where precisely to aim the radiation beams in the treatment of a range of common cancers.
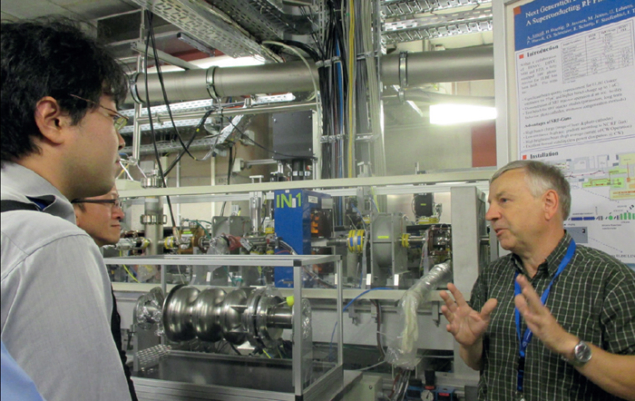
Image credit: C Sutton.
Accelerator-based therapy with carbon ions is coming of age, since the first clinical trials began 20 years ago at the Heavy-Ion Medical Accelerator in Chiba, Japan (CERN Courier June 2010 p22). The experience gained there led to the construction of a pilot for a standard carbon-ion radiotherapy facility at Gunma University, and its successful operation in turn led to projects for two more facilities. In addition, the National Institute of Radiological Sciences is developing a new treatment procedure based on pencil-beam 3D scanning for both static and moving targets. This has been working successfully since May 2011 and will now be used in the Ion-beam Radiation Oncology Centre in Kanagawa.
Accelerators could also be applied in future to address the ongoing shortages in reactor-based supplies of molybdenum-99, which is used in hospitals to produce technetium-99m, a gamma emitter that is important in imaging. Alternative production methods could use conventional or laser-based particle accelerators.
Medical applications are one aspect of a wider industrial and commercial involvement in the field of accelerators that formed the topic of a special session entitled “Engagement with Industry”. Large-scale science projects require collaboration with industry for the “mass production” of large numbers of highly specialized components – 100 superconducting RF modules in the case of the European XFEL project – which presents challenges for both sides. On the other hand, applications such as particle therapy require the industrial development of commercially available accelerators. Another future market could be for electron linacs to provide radiation with photon energy up to 10 MeV for cargo and vehicle inspection. In addition, the traditional industrial exhibition, which took place during the first three days of the conference, attracted exhibitors from 93 companies who occupied 100 booths – the highest number for IPAC to date – to present their high-technology products and services to the delegates.
For many years, the measure of progress for accelerators has been the Livingston curve, but the straight line of the first 50 years has flattened out since the 1980s. One hope, following its proposal in 1979, has been to harness the high electric-field gradients that can be created in plasmas, and here a parallel almost-straight line is emerging on the Livingston plot (see figure above). The technique relies on high-power pulsed lasers or short electron bunches to excite ultra-strong wakefields in plasmas (CERN Courier November 2013 p17). Experiments have produced accelerating gradients from 10 GV/m up to 100 GV/m, and an absolute energy gain for electron beams of up to more than 40 GeV. However, the challenge remains to achieve a beam of useful quality, whether for science or for other applications, and initiatives are under way in various countries to investigate the underlying physics further.
Using the interaction of intense laser beams with a solid target as a means to accelerate protons and ions has a shorter history, following the discovery of such an effect in 2000. For some years the proton energy produced seemed limited to 70 MeV, but recent experiments have shown that the “laser break-out afterburner” mechanism can produce protons with energies up to 130 MeV. Other effort has gone into testing methods for producing useful intense, mono-energetic beams. These systems offer potential for opening up ion-beam physics and neutron science based on short-pulse lasers in universities, and could become ideal compact sources of ion beams for medical applications.
The programme at IPAC’14 highlighted the diverse demands that exist today on accelerator R&D, coming from a variety of fields – neutron sources, synchrotron radiation, medical applications, etc. Accelerator physics and technology is maturing into a research field in its own right and needs well-planned R&D programmes to provide long-term solutions to these requests. In this respect, the field has outgrown its origins in high-energy physics, but the conference ended back at the high-energy frontier, where recent results from the LHC and other facilities have had a significant impact on particle physics. However, outstanding questions remain, and these will continue the drive to higher energies. Projects such as the FCC with which the conference started are among the important options for the future – a future that seems set to see the breadth of accelerator research continue to grow.
• IPAC’14 was organized under the auspices of the European Physical Society Accelerator Group (EPS-AG), the Asian Committee for Future Accelerators (ACFA), the American Physical Society Division of Physics of Beams (APS-DPB) and the International Union of Pure and Applied Physics (IUPAP). For the programme and all of the contributions, see http://accelconf.web.cern.ch/AccelConf/IPAC2014/. In 2015, IPAC will return to North America and take place in Richmond, Virginia.
Participants, prizes and proceedings |
IPAC’14 attracted more than 1200 full-time delegates from 36 different countries from all of the inhabited continents. The attendance of more than 90 young scientists from across the world was made possible through the sponsorship of societies, institutes and laboratories worldwide. Hosted by the Helmholtz-Zentrum Dresden-Rossendorf (HZDR), the conference was supported by the GSI Helmholtz-Zentrum für Schwerionenforschung, the Helmholtz-Zentrum Berlin (HZB) and DESY. Altogether there were 46 invited talks and 51 contributed oral presentations, and 1300 posters were scheduled during lively dedicated sessions at the end of each afternoon. A special student poster session took place during registration, the day before the conference opened. Prizes awarded by the European Physical Society’s Accelerator Group (EPS-AG) for the best student posters were presented later in the week during the special awards session. The prizes went to Eléonore Roussel of PhLAM/CERCLA and Marton Ady of CERN. Lieselotte Obst of HZDR received the EPS student poster prize for a Master’s Thesis student. This year the awards session featured the EPS-AG prizes announced earlier this year (CERN Courier May 2014 p31). Agostino Marinelli of SLAC, Tsumoru Shintake of Okinawa Institute of Science and Technology Graduate University and Mikael Eriksson of the Max-IV Laboratory were all at the conference to receive their prizes and make short presentations about their work. In addition, Juan Esteban Müller of CERN/EPFL received the EPS-AG prize – awarded to a student registered for a PhD or diploma in accelerator physics or engineering, or to a trainee accelerator physicist or engineer in the educational phase of their professional career, for the quality of their work and promise for the future – for his work on a “High-accuracy Diagnostic Tool for Electron Cloud Observation in the LHC based on Synchronous Phase Measurements”. The proceedings of IPAC’14 are published on the JACoW website (www.jacow.org). Thanks to the work of the dynamic team and the careful preparations and guidance of Christine Petit-Jean-Genaz (recently retired from CERN), a pre-press version with close to 1300 contributions was published at mid-day on the last day of the conference. The final version, with the invaluable assistance of Volker Schaa of GSI and chair of JACoW, was published on the JACoW website just three weeks after the conference – another impressive record set by the JACoW collaboration. |