Bringing the LHC to life and steering it beyond its design performance has been a rollercoaster journey for those at the helm, describes Mike Lamont.
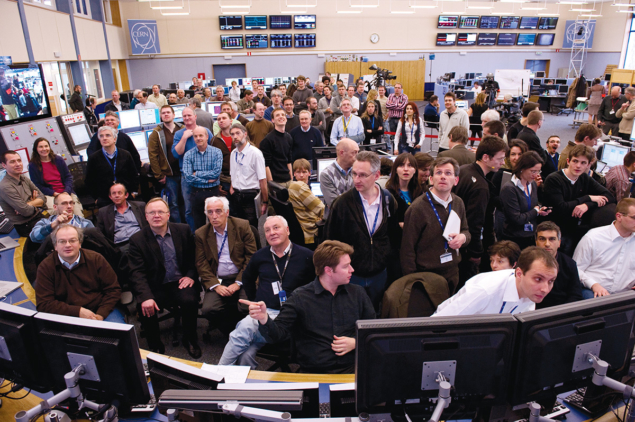
The start-up of the LHC was an exciting time and the culmination of years of work, made manifest in the process of establishing circulating beams, ramping, squeezing and producing the first collisions. The two major events of the commissioning era were first circulating beams on 10 September 2008 and first high-energy collisions on 30 March 2010. For both of these events the CERN press office saw fit to invite the world’s media, set up satellite links, arrange numerous interviews and such. Combined with the background attention engendered by the LHC’s potential to produce miniature black holes and the LHC’s supporting role in the 2009 film Angels and Demons, the LHC enjoyed a huge amount of coverage, and in some sense became a global brand in the process (CERN Courier September 2018 p44).
The LHC is one of biggest, most complex and powerful instruments ever built. The large-scale deployment of the main two-in-one dipoles and quadrupoles cooled to 1.9 K by superfluid helium is unprecedented even in particle physics. Many unforeseen issues had to be dealt with in the period before start-up. A well-known example was that of the “collapsing fingers”. In the summer of 2007, experts realised that the metallic modules responsible for the electrical continuity between different vacuum pipe sections in the magnet interconnects could occasionally become distorted as the machine was warmed up. This distortion led to a physical obstruction of the beam pipe. The solution was surprisingly low-tech: to blow a ping-pong-sized ball fitted with a 40 MHz transmitter through the pipes and find out where it got stuck.
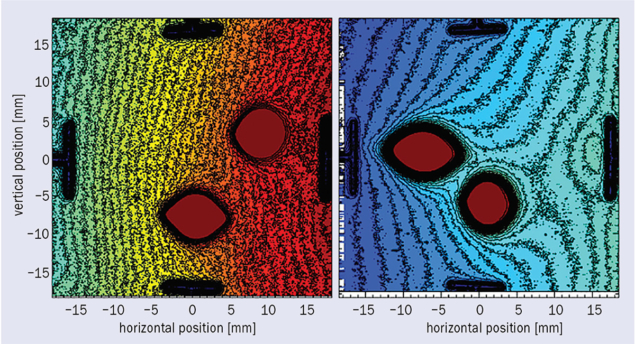
The commissioning effort was clearly punctuated by the electrical incident that occurred during high-current tests on 19 September 2008, just nine days after the success of “first beam day”. Although the incident was a severe blow to CERN and the LHC community, it did provide a hiatus of which full use was made (see A labour of love). The LHC and experiments returned at “an unprecedented state of readiness” and beam was circulated again on 20 November 2009. Rapid progress followed. Collisions with stable beam conditions were quickly established at 450 GeV, and a ramp to the maximum beam energy at the time (1.18 TeV, compared to the Tevatron’s 0.98 TeV) was successfully achieved on 30 November. All beam-based systems were at least partially commissioned and LHC operators managed to start to master the control of a hugely complex machine.
After the 2009 Christmas technical stop, which saw continued deployment of the upgraded quench-protection system that had been put in place following the 2008 incident, commissioning started again in the new year. Progress was rapid, with first colliding beams at 3.5 TeV being established on 30 March 2010. It was a tense day in the control room with the scheduled collisions delayed by two unsuccessful ramps and all under the watchful eye of the media. In the following days, squeeze-commissioning successfully reduced the β* parameter (which is related to the transverse size of the beam at the interaction points) to 2.0 m in ATLAS and CMS. Stable beams were declared, and the high-energy exploitation of the four main LHC experiments could begin in earnest.
Tales from Run 1
Essentially 2010 was devoted to commissioning and then establishing confidence in operational procedures and the machine protection system before starting the process of ramping up the number of bunches in the beam.
In June the decision was taken to go for bunches with nominal population (1.15 × 1011 protons), which involved another extended commissioning period. Up to this point, only around one fifth of the nominal bunch population was used. To further increase the number of bunches, the move to bunch trains separated by 150 ns was made and the crossing angles spanning the experiments’ insertion regions brought in. This necessitated changes to the tertiary collimators and a number of ramps and squeezes. We then performed a carefully phased increase in total intensity. The proton run finished with beams of 368 bunches of around 1.2 × 1011 protons per bunch, and a peak luminosity of 2.1 × 1032 cm–2s–1, followed by a successful four-week long lead–lead ion run.
The initial 50 and 25 ns intensity ramp-up phase was tough going
In 2011 it was decided to keep the LHC beam energy at 3.5 TeV, and to operate with 50 ns bunch spacing – opening the way to significantly more bunches per beam. Following several weeks of commissioning, a staged ramp-up in the number of bunches took us to a maximum of 1380 bunches. Reducing the transverse size of the beams delivered by the injectors and gently increasing the bunch population resulted in a peak luminosity of 2.4 × 1033 cm–2s–1 and some healthy luminosity-delivery rates. Following a reduction in β* in ATLAS and CMS from 1.5 m to 1.0 m, and further gradual increases in bunch population, the LHC achieved a peak luminosity of 3.8 × 1033 cm–2s–1 – well beyond expectations at the start of the year – and delivered a total of around 5.6 fb–1 to both ATLAS and CMS.
2012 was a production year at an increased beam energy of 4 TeV, with 50 ns bunch spacing and 1380 bunches. A decision to operate with tighter collimator settings allowed a more aggressive squeeze to a β* of 0.6 m, and the peak luminosity was quickly close to its maximum for the year, followed by determined and long-running attempts to improve peak performance. Beam instabilities, although never debilitating, were a reoccurring problem and there were phases when they cut into operational efficiency. By the middle of the year another 6 fb–1 had been delivered to both ATLAS and CMS. Combined with the 2011 dataset, this paved the way for the announcement of the Higgs discovery on 4 July 2012. It was a very long operational year and included the extension of the proton–proton run until December, resulting in the shift of a four-week-long proton–lead run to 2013. Integrated-luminosity rates were healthy at around the 1 fb–1 per-week level and this allowed a total for the year of about 23 fb–1 to be delivered to both ATLAS and CMS.
Five phrases LHC operators learned to love

Single-event effects
Caused by beam-induced radiation to tunnel electronics, these were a serious cause of inefficiency in the LHC’s early days. However, the problem had been foreseen and its impact was considerably reduced following a sustained programme of mitigation measures – including shielding campaigns prior to the 2011 run.
Unidentified falling objects
Microscopic particles of the order of 10 microns across, which fall from the top of the vacuum chamber or beam screen, become ionised by collisions with circulating protons and are then repelled by the positively charged beam. While interacting with the circulating protons they generate localised beam loss, which may be sufficient to dump the beam or, in the limit, cause a quench. During the first half of 2015 they were a serious issue, but happily they have subsequently conditioned down in frequency.
Beam-induced heating
This is where regions of the LHC near the beam become too warm, and has been a long-running issue. Essentially, all cases have been local and, in some way, due to non-conformities either in design or installation. Design problems have affected the injection protection devices and the mirror assemblies of the synchrotron radiation telescopes, while installation problems have occurred in a low number of vacuum assemblies. These issues have all been addressed and are not expected to be a problem in the long term.
Beam instabilities
This was an interesting problem that occasionally dogged operations. Operations with 25 ns bunch spacing and lower bunch population have meant that intrinsically instabilities should have been less of an issue. However, high electron cloud (see “Electron cloud effects”) also proved to be a driver and defence mechanisms were deployed in the form of high-chromaticity, high-octupole field strength, and the all-important transverse damper system.
Electron cloud effects
These result from an avalanche-like process in which electrons from gas ionisation or photo-emission are accelerated in the electromagnetic field of the beam and hit the beam-chamber walls with energies of a few hundreds of eV, producing more electrons. This can lead to beam oscillations and blow-up of the proton bunches. “Scrubbing”, the deliberate invocation of high electron cloud with beam, provides a way to reduce or suppress subsequent electron cloud build-up. Extensive scrubbing was needed for 25 ns running. Conditioning thereafter has been slow and the heat load from electron cloud to cryogenics system remained a limitation in 2018.
To Run 2 and beyond
In early 2015 the LHC emerged from “long-shutdown one”. The aims were to re-commission the machine without beam following major consolidation and upgrades, and from a beam perspective to safely establish operations at 6.5 TeV with 25 ns bunch spacing and around 2800 bunches. This was anticipated to be more of a challenge than previous operations at 4 TeV with 50 ns beams. Increased energy implies lower quench margins and thus lower tolerance to beam loss, with hardware pushed closer to maximum with potential knock-on effects to availability. A 25 ns beam was anticipated to have significantly higher electron-cloud effects (see “Five phrases LHC operators learned to love” box) than that experienced with 50 ns; in addition, there was a higher total beam current and higher intensity per injection. All of these factors came into play to make 2015 a challenging year.
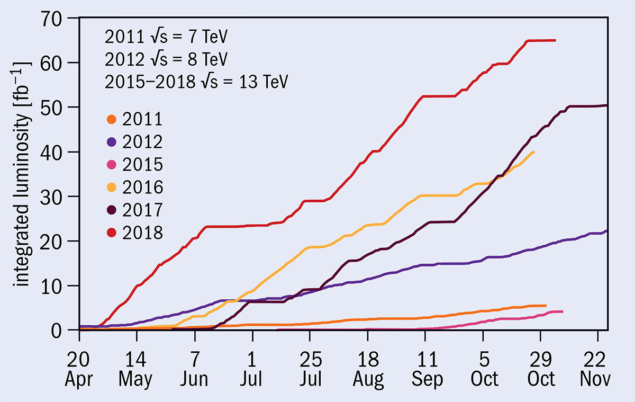
The initial 50 and 25 ns intensity ramp-up phase was tough going and had to contend with a number of issues, including earth faults, unidentified falling objects, an unidentified aperture restriction in a main dipole, and radiation affecting specific electronic components in the tunnel. Nonetheless, the LHC was able to operate with up to 460 bunches and deliver some luminosity to the experiments, albeit with poor efficiency. The second phase of the ramp-up, following a technical stop at the start of September, was dominated by the electron–cloud-generated heat load and the subsequent challenge for the cryogenics, which had to wrestle with transients and operation close to their cooling power limits. The ramp-up in number of bunches was consequently slow but steady, culminating in the final figure for the year of 2244 bunches per beam. Importantly, the electron cloud generated during physics operations at 6.5 TeV served to slowly condition the surface of the beam screens in the cold sectors and so reduce the heat load at a given intensity. As time passed, this effect opened a margin for the use of more bunches.
The overall machine availability remained respectable with around 32% of the scheduled time spent in “stable beams” mode during the final period of proton–proton physics from September to November. By the end of the 2015 proton run, 2244 bunches per beam were giving peak luminosities of 5.5 × 1033 cm–2s–1 in the high-luminosity experiments, with a total integrated luminosity of around 4 fb–1 delivered to both ATLAS and CMS. Levelled luminosities of 3 × 1032 cm–2s–1 in LHCb and 5 × 1030 cm–2s–1 in ALICE were provided throughout the run.
A luminous future
Following an interesting year, 2016 was the first full year of exploitation at 6.5 TeV. The beam size at the interaction point was further reduced (β* = 0.4 m) and the LHC design luminosity of 1034 cm–2s–1 was achieved. Reasonable machine availability allowed a total of 40 fb–1 to be delivered to both ATLAS and CMS. 2017 saw a further reduction in beam size at the interaction point (β* = 0.3 m), which, together with small beams from the injectors, gave a peak luminosity of 2.2 × 1034 cm–2s–1. Despite the effects of an accidental ingress of air into the beam vacuum during the winter technical stop, around 50 fb–1 was delivered to ATLAS and CMS.
Not only can a 27 km superconducting collider work, it can work well!
2018 essentially followed the set-up of 2017 with a squeeze to β* = 0.3 m in ATLAS and CMS. The effects of the air ingress lingered on, limiting the maximum bunch intensity to approximately 1.2 × 1011. Despite this, the peak luminosity was systematically close to 2 × 1034 cm–2s–1 and around 63 fb–1 was delivered to ATLAS and CMS. Somewhat more integrated luminosity was possible thanks to the novel luminosity levelling strategy pursued. This involved continuous adjustment of the crossing angle in stable beams, and for the first time the LHC dynamically changed the optics in stable-beams mode, with β* reduced from 0.30 to 0.27 to 0.25 m while colliding. The year finished with a very successful lead–ion run, helped by the impressive ion delivery from the injectors. In December 2018 the machine entered long-shutdown two, recovery from which is scheduled in 2021.
It is nearly 12 years since first beam, and 10 since first high-energy operations at the LHC. The experience has shown that, remarkably, not only can a 27 km superconducting collider work, it can work well! This on the back of some excellent hardware system performance, impressive availability, high beam quality from the injectors and some fundamental operational characteristics of the LHC. Thanks to the work of many, many people over the years, the LHC is now well understood and continues to push our understanding of how to operate high-energy hadron colliders and to surpass expectations. Today, as plans for Run 3 take shape and work advances on the challenging magnets needed for the high-luminosity LHC upgrade, things promise to remain interesting.