After 50 years in waiting, fixed-field alternating-gradient accelerators are at last being built – for a wide variety of applications. Michael Craddock reports on the current status.
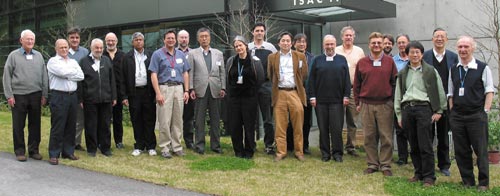
Fixed-field alternating-gradient (FFAG) accelerators, which were intensively studied in the 1950s and 1960s but never progressed beyond the model stage, have in recent years become the focus of renewed attention. Two proton machines have already been built and three more, plus an electron FFAG and a muon phase rotator, are under construction. A variety of designs are also under study for the acceleration of protons, heavy ions, electrons and muons, with applications as diverse as cancer therapy, industrial irradiation, driving subcritical reactors, boosting high-energy proton intensity and neutrino production. These advances have been underpinned by a series of international workshops, the first being held at CERN in 2000, with subsequent meetings at KEK (twice), LBNL, BNL and TRIUMF. The next workshop will again be held at KEK, in October 2004.
With their fixed magnetic fields, modulated radiofrequency (RF) and pulsed beams, FFAGs operate just like synchrocyclotrons – in fact they bear the same relation to classic synchrocyclotrons as isochronous ring cyclotrons (such as at PSI, IUCF, RIKEN and so on) do to the classic Lawrence cyclotron: the central region has been removed and the magnet broken into radial or spiral sectors to provide edge and strong focusing.
The fixed magnetic field leads to a spiral orbit, so the vacuum chamber and magnets tend to be larger than for a synchrotron, but the repetition rate (and hence beam intensity) can be much higher, as it is set purely by RF considerations. High repetition rate and large momentum acceptance are the two features where FFAGs offer advantages over synchrotrons, and it is applications needing one or both of these features that have driven the current surge of interest.
Following the discovery of alternating gradient (AG) focusing in 1952, FFAGs were proposed independently by Tihiro Ohkawa in Japan, Keith Symon in the US and Andrei Kolomensky in the USSR. The most intensive studies were carried out by Symon, Donald Kerst and others at MURA (the Mid-western Universities Research Association) in Wisconsin, and culminated in the construction and successful testing of electron models of radial-sector and spiral-sector designs. But the proposals for proton FFAGs were not fun-ded at that time, nor in the 1980s when 1.5 GeV machines were proposed by the Argonne and Jülich laboratories as spallation neutron sources.
Scaling FFAGs
In order to avoid the slow crossing of betatron resonances associated with conventional low energy-gain per turn, all the FFAGs constructed so far have been based on the “scaling” principle. This means that the orbit shape, optics and betatron tunes are kept fixed, independent of energy, just as in synchrotrons. One implication of this is that the magnets must be built with constant field index (logarithmic gradient). In the case of spiral-sector designs it also implies a constant spiral angle. The need for both strong gradient and high spiral angle (to achieve adequate focusing) makes the spiral magnet design very challenging.
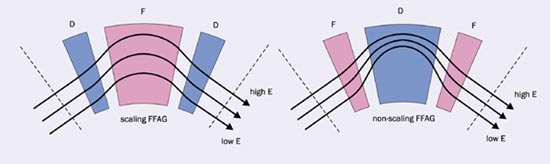
For radial-sector designs the focusing (F) and defocusing (D) gradient magnets must have equal and opposite fields (but different lengths) to give enhanced “magnetic flutter” (rms field variation) and strong edge as well as AG focusing. The two proton FFAGs recently built by Yoshiharu Mori’s group at KEK are of this type. The 1 MeV POP (proof of principle) FFAG has eight sectors, each consisting of a “DFD” radial-sector triplet. It came into operation in 2000 and measurements on the beam have provided valuable confirmation of its predicted behaviour.
The larger 150 MeV FFAG at KEK is a prototype for proton therapy and neutron production. It has 12 sectors, also DFD, with the orbit radius increasing from 4.4 to 5.3 m. Beam from the 12 MeV cyclotron injector has been accelerated to full energy and the extraction system is currently being commissioned.
A key technical innovation by Mori’s KEK group has been the use of Finemet metallic alloy for rapid modulation of the RF frequency at repetition rates higher than are practical with ferrite (250 Hz in the larger machine, with a 1.5 to 4.6 MHz sweep). This material has two advantageous properties: high permeability, permitting short cavities and a high effective accelerating field; and lossiness, making the quality factor very low (Q ~ 1) and so allowing acceleration over a wide frequency range without any need for active tuning.
A 150 MeV FFAG of the same design is also being installed at the Kyoto University reactor, in collaboration with Mitsubishi, to test accelerator-driven sub-critical reactor operation. Two further FFAGs act as injector (a 2.5 MeV betatron with eight spiral sectors) and booster (20 MeV with eight radial sectors). Initially the repetition rate will be 120 Hz, yielding a 1 µA beam, and then later 1 kHz, providing 100 µA.
For heavy-ion therapy the National Institute of Radiological Sciences at Chiba in Japan, home of the Heavy Ion Medical Accelerator (HIMAC), has designed a three-stage FFAG, comprising rings operating at 6, 100 and 400 MeV/u. The largest, with 12 radial sectors, has a circumference of 70 m. The complex is designed to operate at 200 Hz and provide a beam of 2 x 109 C+ions/s. Mitsubishi is also designing heavy-ion FFAGs for therapy, but of spiral-sector design. In this case a 12-sector booster (3.5-4.0 m radius) would accelerate C+ ions to 62 MeV/u, or protons to 230 MeV. The 16-sector main ring (6.6-7.2 m radius) would take the C+ to 400 MeV/u.
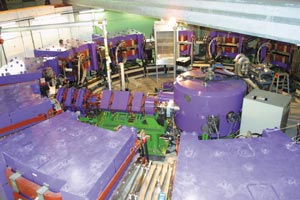
Another Mitsubishi project is the construction of a 1 MeV electron FFAG betatron as a scaled-down prototype for industrial irradiation, CT scanning and radiation therapy. This machine, appropriately named “Laptop”, has five spiral sectors, an overall diameter of 10 cm and a magnet weighing all of 2.8 kg!
Muon FFAGs
FFAGs are also of interest for muons at both low and high energies. PRISM (Phase-Rotated Intense Slow Muon Source), based on a 10-cell “DFD” radial-sector FFAG of 6.5 m radius, is under construction at RCNP Osaka for eventual installation at J-PARC. It will collect 5 ns wide bunches of muons at 68 MeV/c ± 30% and use a sawtooth RF field to rotate them in phase space, reducing the momentum spread to ± 3%. With a repetition rate of 100-1000 Hz the muon intensity will be 1011-1012/s, making possible ultra-sensitive studies of rare muon decays. It is also planned to use PRISM for ionization cooling of muons. Another proposal for ionization cooling comes from Al Garren at UCLA and Harold Kirk and Stephen Kahn at Brookhaven, who suggest using a small 12-sector gas-filled FFAG with superconducting magnets (96 cm radius) for cooling muons of 250 MeV/c ± 30%.
The KEK group’s most ambitious plan is to build a neutrino factory at J-PARC based on a sequence of four muon FFAGs with top energies of 1, 3, 10 and 20 GeV. The largest would have a radius of 200 m (with a total orbit spread of 50 cm) and consist of 120 cells, each containing a superconducting DFD triplet. Most of the cells would also contain RF cavities to provide an overall energy gain of around 1 GeV per turn, restricting the losses through muon decay to 50% overall. The use of low-frequency RF (24 MHz) keeps the buckets wide enough to contain the phase drift occurring as the orbit expands. A major advantage of FFAGs over linacs – either single or recirculating – is that their large acceptance obviates the need for muon cooling or phase rotation. There also turn out to be significant cost savings.
Non-scaling FFAGs
The rapid acceleration that is essential for muons allows betatron resonances no time to damage beam quality. The scaling principle can therefore be relaxed, the betatron tunes allowed to vary and lattices explored for properties that are favourable to muons. In particular, in 1999 Carol Johnstone at Fermilab showed that it would be very advantageous to make the positive bend D and the negative F (so that their fields decrease outwards rather than increasing as demanded by scaling), with the Fs weaker as well as shorter than the Ds. The circumference could be shortened; the radial orbit spread reduced, allowing the use of smaller vacuum chambers and magnets; and the orbit length made to pass through a minimum at mid-energy (instead of rising monotonically), thus reducing the variation in orbit time with energy – a vital consideration since there is no time for RF frequency modulation. Moreover, constant field gradients could be used (rather than constant field index), simplifying the magnet design and rendering non-linear resonances harmless.
Lattices along these lines have been developed by Johnstone at Fermilab, by Scott Berg, Ernest Courant, Dejan Trbojevic and Robert Palmer at Brookhaven, by Eberhard Keil at CERN and Andy Sessler at LBNL, and by Shane Koscielniak at TRIUMF.
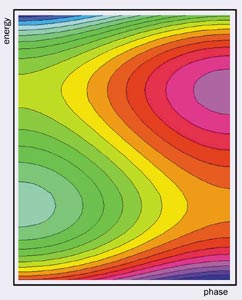
The latest results from an ongoing cost-optimization study by Berg and colleagues favour the use of linacs up to 2.5 GeV, followed by 2.5-5.0, 5-10 and 10-20 GeV FFAGs. The main ring, to be composed of around 100 doublet or FDF triplet cells, would have a circumference of about 700 m, with orbit lengths varying by only 20 cm. With the orbit time first falling and then rising, Koscielniak and Berg have shown that by exceeding a critical RF voltage an acceleration path can be created that stays close to the voltage peak (crossing it three times), snaking between neighbouring buckets (rather than circulating inside them). By using high-field superconducting 200 MHz cavities it should be possible to accelerate from 10 to 20 GeV in 16 turns, with a decay loss of 10% (25% in the three rings). In order to demonstrate the novel features of such a design – particularly acceleration outside buckets and the crossing of many integer and half-integer resonances – the construction of a 10-20 MeV/c electron model is being considered.
Grahame Rees from the Rutherford Appleton Laboratory is proposing a very different scheme for an 8-20 GeV muon ring using 0BFDFB0 cells in which the F and D fields are profiled to make each of the 16 orbits exactly isochronous, allowing acceleration at peak RF voltage throughout. The price paid is a somewhat larger circumference of 1255 m. This is one of several European FFAG studies that are being coordinated through Beams for European Neutrino Experiments (BENE).
Yet another non-scaling approach has been taken by Alessandro Ruggiero at Brookhaven in a design for a 1.5 GeV proton FFAG to replace the laboratory’s Alternating Gradient Synchrotron (AGS) Booster, or for high-power applications up to 4 MW. Here acceleration is relatively slow (> 1000 turns) so that resonances must be avoided. The tune is therefore kept essentially constant by using a non-linear field profile for which the changes in gradient balance those in flutter, while the non-scaling virtue of low dispersion is retained by using FDF cells with stronger D magnets than F magnets. The 136-cell FFAG, to be located in the AGS tunnel, would accelerate 1014 protons per pulse at 2.5 Hz, providing a 40 µA beam.
With this wide variety of new ideas and projects, it seems that FFAGs have at last come into their own. Rather than merely a historical curiosity from the mid-20th century, they are now revealed as a vital answer to the needs of the 21st.
Further reading
For more about the workshop at TRIUMF, see www.triumf.ca/ffag2004.